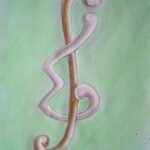
Mural paintings in tombs are always facing protection problems in the process of display. In order to achieve the necessary balance between fresco protection and display, this paper discusses the application scenario and implementation steps of utilizing digital twin technology to protect frescoes, and builds a fresco protection display system. Focusing on gesture interaction, this paper uses Kinect interactive device to realize the recognition of human gestures. The average recognition speed of this paper’s method is about 0.02s, and it has high recognition accuracy under different angles and depths, and different gesture movement trajectories. The designed gesture virtual interaction system can improve the satisfaction of visiting the tomb murals and realize the balance between the protection and display of murals.
Cross-language text categorization techniques can achieve more efficient localization and use of text data in multilingual languages by overcoming the differences between different languages. In this paper, firstly, by combining cross-language word vectors and adversarial training, support vector machines are utilized to improve the alignment effect of English-Chinese cross-language words and sentences in the feature space, and to achieve higher quality English-Chinese cross-language text classification. Then the variational mechanism is combined with multi-task learning to align the potential semantic space of multimodal data, maintain the domain invariance of different modal data representations, improve the generalization ability of the model, and ensure the consistency of the variational machine translation training process and the prediction process. The two are combined to construct a hybrid variational multimodal machine translation model based on semantic alignment, experimentally validate the effect of the text categorization algorithm on datasets such as Multi30k, and examine the quality of English-Chinese and Chinese-English translations. In the experiments, it is found that on the MSCOCO dataset, the BLEU of English to Chinese and Chinese to English of this paper’s model is 61.26 and 60.15 respectively, and the translation quality is significantly better than the baseline model. The model achieved the best results in all 3 actual translation tasks. And compared with the complete model, the translation performance of different removal cases in the ablation experiments are decreased, which verifies the effectiveness of the model of this paper as a whole and different components. The method in this paper can effectively reduce the feature differences between different languages, and has important practical application value for solving cross-language text categorization and machine translation problems.
As a small-scale power generation and distribution system, microgrid, by virtue of its high efficiency and clean power generation, has been taken by scholars around the world as a key research object for the sustainable development of national energy. Taking microgrid as the main research object, this paper explores the construction of power load identification model and optimization of scheduling capacity of microgrid. The improved Least Squares Support Vector Machine (LS-SVM) algorithm is used to construct the power load identification model, which realizes the accurate prediction of power load data. The optimal scheduling model of the microgrid is constructed based on the nonlinear planning method, and the co-evolutionary genetic algorithm (DCGA) with the improved difference strategy is used to solve and find the optimal model.The curve of the predicted value of the power load of the LS-SVM is basically fitted to the curve of the real value, and its prediction of the power load is more accurate than that of the BP neural network model. The daily running costs of the genetic algorithm, CCGA algorithm and DCGA algorithm are 1750.34 yuan, 1730.59 yuan and 1709.83 yuan, respectively. The daily running cost of the improved DCGA algorithm in this paper is 1763.59 yuan, which is reduced by 2.31% and 1.20% compared with the genetic algorithm and co-evolutionary genetic algorithm, respectively, and the DCGA algorithm has the fastest convergence speed, which indicates that it has the strongest ability to search for optimization, and it can effectively reduce the operating cost of microgrids, and it has a high practical value.
Civil engineering cracks are located in complex and diverse environments, often interfered by a variety of external background factors, and crack detection faces various challenges. This paper proposes an improved YOLO v8s-WOMA network, which mainly introduces the ODConv module, C2f-MA module and WIoU loss function to solve the problem of many interferences and low accuracy of civil engineering crack identification in complex background. At the same time, combined with the determination criteria of civil engineering crack damage, the BP neural network is trained to evaluate the damage degree of cracks. Experimental validation is carried out on the CBP dataset dataset to compare the algorithm of this paper and with the existing target detection algorithm. The experimental results show that the proposed YOLO v8s-WOMA network outperforms other algorithms in several target detection performance evaluation indexes, and this paper’s algorithm has the highest mAP value, F1 value, and accuracy value, which are 90.5%, 90.3%, and 89.6%, respectively. The width error of the actual bridge crack detection does not exceed 0.1mm, the length error does not exceed 20mm, and it can accurately determine the degree of civil engineering damage. It shows that the model in this paper can effectively deal with the interference of complex background, accurately detect bridge cracks, and can basically meet the practical application of civil engineering crack detection.
In this paper, the embedding vectors are obtained by Bert coding, and then the obtained embedding vectors are adaptively fused with features to realize legal text classification by a classifier, on the basis of which a multi-label text classification model (AFDAM) is proposed to capture the target words in a sentence. At the same time, the pre-trained continuous bag-of-words representation (CBOW) is used to initialize the vector representation of the label information, and then these label information is adaptively fused with the feature information of the text, which effectively promotes the multi-label legal text classification, and accelerates the development of informationization and intelligence in the legal field. The results show that the text feature enhancement module has the most prominent impact on the text classification effect, and its accuracy on the three datasets is improved by 0.46%-1.19%. In addition, the introduction of target vectors and text expansion also gained 0.54%-1.7% and 0.59%-1.53% and 1.08% increases in model accuracy, respectively. In addition, the addition of offense and statute information can significantly improve the prediction of sentence length, and the statute information improves the results more significantly than the offense information. And the classification effect of the AFDAM model proposed in this paper increased by 0.1453-0.257 than the other five models.
Quality management is one of the factors determining the running level of a university, so it is necessary to evaluate university management scientifically and comprehensively. In this paper, a university management evaluation index system is constructed and optimized with reference to the multi-factor decision tree technology, and the cause degree and centrality degree are calculated through the DEMATEL method model, the causality diagram is established, and the ISM hierarchical structure analysis is subsequently carried out. The management influences with high centrality degree are service ability, student quality, employment, school size and presidential leadership. Through the results of the reachable matrix, four levels of college management influencing factors are divided, and it is found that the fundamental factors affecting the management level of colleges and universities are concentrated in the socio-economic and cultural level, the deeper factors are mainly the school’s own scale, funds and teachers and students, and the leadership quality and service ability are the superficial factors. Therefore, the improvement of university management evaluation system can be carried out with reference to the above levels and indicators.
With the rapid development of social networks, the powerful interactive function of social networks and the high degree of user participation make the information generated in large quantities and spread rapidly, which also provides a dissemination path for the mass dissemination of Marxism. The article analyzes the overall structure of complex social networks and node centrality indexes for the overall topological characteristics of the networks, in order to further analyze the information dissemination characteristics of social networks for the mass dissemination of Marxism. Subsequently, a social network information dissemination model based on SIR is established according to the specific structural characteristics and dissemination modes of the social network, and the experimental test of the information dissemination effect is carried out. Finally, a Marxist mass communication strategy is proposed based on the experimental results. In the experiments on the effect spreading of node information, the nodes numbered 4, 12, and 20 have the strongest information spreading effect, with the number of nodes infected exceeding 30, and the corresponding number of interaction activities are 575, 511, and 663.This suggests that individuals within the aggregated group can build trust with the group members by participating in frequent interactions to improve the effect of information spreading. The development and dissemination of Marxist mass social networks cannot be separated from a series of measures such as a sound social network regulatory system.
This paper introduces a multilayer Bayesian model based on probabilistic and Bayesian inference models to infer discourse hierarchical features in the English corpus at both intra-individual and inter-individual behavior levels. Based on the existing English corpus observation data, the Bayesian method is used to organically combine the prior knowledge and the observed English corpus discourse hierarchical features data, derive and incorporate the posterior probability distribution of many uncertain information target variables, and obtain the discourse hierarchical features of excellent English teachers in the English corpus, which provides more valuable information for educational management. For the emotional features of different teachers’ classroom discourse, the level of “emotionally full” is higher than that of “emotionally depressed”, which indicates that the classroom emotions of excellent teachers are more full, demonstrating the discourse hierarchy features of excellent English teachers. The Bayesian estimation has excellent estimation accuracy and explains the discourse hierarchy of teachers in the English corpus well.
Apriori algorithm is a classic frequent itemset mining algorithm, but it has the problems of more time consumed by the self-connection process and high overhead of conversion between memories. In order to improve the frequent itemset mining effect of Apriori algorithm, this paper improves the existing adaptive genetic algorithm by using the average population fitness and fitness value discretization, and improves the Apriori algorithm by using the optimized genetic algorithm, so as to solve the strong association rules. Compared with the traditional Apriori algorithm, the algorithm in this paper has less time overhead and improves 2.4%, 2.4%, and 2.7% on average in recall, accuracy, and F1 value. On the Accidents and Retail datasets, the improved Apriori algorithm is faster than the NSFI algorithm by 6.12% and 13.52% on average, reducing the computational complexity. Using the improved algorithm to analyze the characteristics of cross-provincial migrants, it is found that the migrant population is younger in age, with lower education level, mostly of agricultural household registration, and mostly of Han nationality, which verifies the practical application value of the improved algorithm.
The physical and mechanical properties of the rock body at the foot of the slope are prone to deterioration under water-rock action, which affects the stability of the slope body. Accurate understanding of the damage mechanism of anticlinal rocky slopes in reservoir area under the condition of deterioration of the rock body at the foot of the slope is the key to the reasonable evaluation of stability. In this paper, the main lithological characteristics of the anti-dipping rocky slopes in the reservoir area and the distribution characteristics of slope height, slope angle and inclination angle of the rock layer are investigated as the research object, and the deformation and damage characteristics and laws of the rock body are obtained. Numerical simulation of anticline slopes was carried out using GDEM mechanical analysis software based on the discrete element method of continuous medium mechanics. It is found that the upper and middle parts of the slope where the invert body is located in the studied engineering example have deep tensile cracks and shallow surface block tipping damage, while the middle and lower parts show deep bending deformation, and there is a gradual transition zone in the contact between the deformed rock layer and the bedrock. As the distance between the cave and the basement increases, the rock layers gradually tilt towards the Yellow River from the north-east to the north-west, with the rock layers at the base of the cave tilting between 340° and 350°. The inclination of the rock layer in the example slope is 76°, and the main rupture surface of the slope, i.e. the location of the largest bending moment of the rock layer, has a small inclination angle with the horizontal plane. The slope angle is 48°, and the sum of the angles of the slope angle and the inclination angle of the rock layer is obviously larger than 117°, and the slope will be deformed and damaged, which is consistent with the value of the conditions for the slope to be deformed and damaged.
Teachers’ educational decision-making behavior is a deep factor affecting the quality of teaching and has a guiding role in the whole process of teaching activities. In this paper, lagged sequence analysis is used to focus on comparing the differences in multi-objective educational decision-making behaviors between backbone teachers and novice teachers. At the same time, a collaborative filtering recommendation algorithm based on improved cosine similarity combining teacher users and teaching resources is designed to achieve personalized teaching resources recommendation for teachers. And the personalized teaching path for teachers was designed by combining the characteristics of teachers’ educational decision-making behaviors. In terms of static decision-making behavior, backbone teachers pay more attention to cognitive decision-making, while novice teachers pay more attention to procedural decision-making. In terms of dynamic decision-making behavior, backbone teachers’ decision-making strategies are more balanced and diverse and goal-focused than novice teachers. The personalized teaching path of this paper is much better than traditional teaching methods in actual teaching experiments, and there is a highly significant difference between the pre and post-test scores of students in the experimental group using the path (p=0.000<0.01), and teachers are more satisfied with the accuracy of the resource recommendation and the teaching effect of the path. The personalized teaching path designed in this paper helps teachers' educational decision-making in teaching and provides a feasible implementation path for personalized teaching.
This paper follows the principle of construction of evaluation index system to formulate the evaluation index system of teaching quality of college courses, which is mainly composed of 5 first-level indexes and 25 second-level indexes, and in addition, the real assessment data of ten students of a 985, 211 college on teachers’ teaching quality assessment is taken as the main source of data for this study. The combined algorithm of hierarchical analysis and fuzzy comprehensive evaluation is used to construct a university course teaching quality assessment model, and the model is analyzed by example verification. The comprehensive evaluation scores of the secondary indicators of the university’s course teaching quality are (2.1781, 2.879, 2.1934, 1.7756, 0.9739), and based on the principle of maximum affiliation degree, it is concluded that the students’ grade of the university’s course teaching quality is good (2.879), and the results are in line with the university’s actual course teaching, and at the same time, it is proved that the model of this paper has an excellent application effect.
In recent years, with the increasing psychological pressure on students, psycho-pedagogical methods have been highly emphasized. This article takes students’ multimodal emotion recognition as a research perspective. The article firstly studies the unimodal emotion recognition methods of expression, text and speech respectively. Then it proposes a multimodal emotion recognition algorithm based on dual-attention mechanism and gated memory network, and then conducts emotion recognition experiments to validate this paper’s method. The article further proposes an intervention pathway to further assist in solving students’ mental health problems by designing a virtual reality mental health intervention system. Using the method of this paper in Multimodal database unimodal emotion recognition experiments, found that the network of the model used in this paper has better experimental results, which verifies the effectiveness of the method of this paper, and the accuracy rate of emotion recognition is 60.65%. After testing the mental health level of 8000 students in a school, it was found that the number of hypermodality and the screening rate were low except for the high score of compulsion, from which it can be concluded that the students in our school are in good mental health as a whole after applying the method of this paper.
Knowledge mapping, as an emerging knowledge management tool, provides a new perspective of knowledge learning for physical education teaching. In this study, knowledge mapping is introduced into physical education teaching, and a comprehensive physical education knowledge map is constructed by integrating the teaching resources and contents of physical education teaching and utilizing related techniques such as knowledge extraction and knowledge fusion. The method of fusion of sports knowledge graph is also proposed, including three parts: graph approximation, similarity calculation, and subgraph fusion. Finally, the constructed knowledge graph is practically applied, and a recommendation model based on sports knowledge graph and neural network is constructed to realize the sports teaching application of intelligent educational knowledge graph. The entity recognition module optimized the recognition accuracy rate on the objective existence entities of sports by 1.45%, and the relationship extraction module outperformed AGGCN in all three indicators. The training method of this paper is better than the MICT sports training method in improving students’ cardiorespiratory capacity and flexibility quality. The improvement of students’ 800m running performance under this paper’s training program is 0.13min more than that of MICT.It is proved that the sports course recommendation model based on knowledge graph and neural network provides a reference for the management and application of knowledge data in physical education, with a view to promoting the progress in the field of intelligent education.
The assessment of economic quality is of great significance in grasping the state of national economic development at a macro level. This paper focuses on exploring the assessment methods of economic quality and introducing deep learning models to improve the shortcomings of the traditional economic quality assessment in the assessment process. The economic quality assessment system is constructed from five dimensions, including economic vitality, and the MIV indicator values are improved by combining set-pair analysis and generalized regression neural network, so as to realize the automatic screening of economic quality evaluation indicators. According to the screening results of the indicators, the hierarchical analysis method is used to assign weights to the indicators, and the comprehensive index of economic quality is measured based on the results of the assignment.From 2012 to 2022, the economic quality of the 30 provinces in China shows an upward trend as a whole, and the comprehensive index of economic quality in 2022 is 0.90, which is an increase of 52.54% compared with that in 2012. The assessment results are consistent with the actual results, indicating that the method of this paper can effectively complete the measurement and assessment of the economic quality index, which is important for the study of economic quality.
The article builds a simulation system based on human physiological parameters, collects human physiological data through the human physiological model, and simulates human physiological signals. The load adaptability of trainers to aerobics training was explored by studying the changes in the SI values of T-lymphocytes of the subjects’ bodies during aerobics training. SPSS and independent samples t-test were used to analyze the exercise data of the experimental group and the control group, so as to verify whether the aerobics training has a good exercise effect. At Week 0, the SI values of T lymphocytes in the immediate post-exercise group and the 3-hour recovery group after exercise were 0.88, 0.61 and 0.70, respectively. In Week 2, it dropped to 0.34 and 0.49, respectively. At Week 6, the SI values of lymphocytes in the two groups were 0.60 and 0.30, respectively. The SI values of T lymphocytes in Week 0, Week 2, Week 4 and Week 6 in the quiet group were 0.88, 0.48, 0.80 and 0.50, respectively. Before the experiment, there was no significant difference between the experimental group and the control group in terms of exercise effect, and after the experiment, a significant difference was produced, and the exercise effect of the experimental group far exceeded that of the control group. The experimental group’s exercise effect improved by 6.43, 5.13, 6.91, 6.38, and 5.80 points on each of the five dimensions, a significant difference. The control group, on the other hand, remained essentially unchanged.
In today’s rapid development of information technology and big data technology, consumer behavior is undergoing a profound transformation. This study focuses on the decision-making stage of consumer journey, selects indicators based on webpage click stream data, improves the K-means algorithm, and realizes the identification of consumer journey nodes using the binary K-means algorithm. Based on the review recommendation scenario, from the perspective of consumer decision-making journey, we introduce the “attention-attitude-understanding-purchase intention” stage-based decision-making model, apply it to the model design of deep learning, and combine the attention mechanism and co-attention mechanism to propose a product recommendation method based on online reviews. The results show that consumers in clusters 1-4 are in the consumer journey nodes of attention, understanding, attitude, and purchase intention, respectively. The product recommendation model exhibits better recommendation accuracy and time efficiency, with accuracy improved by 18.72%~67.12% and time reduced by 8.39%~62.03% over the comparison method. This paper realizes the innovation of deep learning method with the support of consumer behavior theory, and improves the methodological technical support for accurate online marketing strategy.
From the perspective of artificial intelligence (AI), this paper explores the application and impact of cluster analysis in the criticism of narrative ethics in Chinese new century literature. Utilizing AI paper processing technology, a large amount of literary text data is quickly obtained and processed, and a knowledge map of narrative literary works is constructed. Meanwhile, a clustering algorithm is used to divide the keywords of literary works into cluster classes to improve the efficiency of rapid literary analysis. The regression model is used to evaluate the effect of the cluster analysis method in the AI perspective on the ethical criticism of literary narratives. The accuracy, recall, and F1 value of the two AI techniques selected in this paper in the classification of literary text themes, keywords, and emotions are 85% to 90%, which is higher than the comparison methods, and combined with the clustering algorithm, the keyword categories of the literary text can be obtained quickly and precisely. In addition, by constructing a knowledge graph, this paper can help users grasp the character relationships in literary texts more clearly and assist in ethical criticism. The investigators are highly satisfied with the method of this paper, the average rating of each dimension is between 4.09 and 4.7, and the method has a significant contribution to the effect of ethical criticism of literary narratives.
Generative artificial intelligence, as a new technology paradigm, has received more and more attention for its powerful generative ability and wide application prospects. Especially in automated control systems, the application of technology based on generative artificial intelligence is gradually becoming a hot spot of research. In this paper, the generative AI automation control system is divided into four levels: input layer, processing layer, instruction generation and control execution layer, and combined with dual encoders, the attention model of multilingual to semantic expression is constructed. Two-dimensional variables are selected to construct a fuzzy PID control system to realize automation control for generative AI system. Comparing the control effects of fuzzy control PID and classical PID, the average errors of the two systems are 1= , 2= respectively. The maximum overshoot and rise time are 9% and 0.08 s, 5% and 0.04 s. The fuzzy PID control effect is more accurate, and at the same time improves the dynamic performance of the system. Analyze the implementation effect on the innovative service application of generative artificial intelligence. Comparing the overall recognition effect of the control system B proposed in this paper, and the two systems with reference to A, their overall recognition effect indexes are 0.94755 and 0.87211, respectively, and the fuzzy PID control system plays an auxiliary enhancement role in the contextual feature recognition of translation services in the intelligent library.
The legal positioning of blockchain technology applied to evidence and its attributes are the basis for its evidence review and rule design. This paper starts from analyzing the evidence attributes of blockchain electronic data, combines relevant regulations and judicial interpretations, and clarifies the legal effect of blockchain electronic data. Combined with the judicial application of blockchain evidence at home and abroad, it points out the specialized review rules of blockchain evidence. Obtain the blockchain access evidence process, and propose the block file storage method based on RS code as well as the decryption outsourcing attribute-based encryption scheme with the same sub-policy to improve the CP-ABE encryption scheme. Explore the rules for blockchain deposits and clarify the rules and institutional value of blockchain deposits for admissibility. Analyze the theoretical and practical operational performance of the improved attribute-based encryption algorithm. Optimize the evidence storage capacity of blockchain, and analyze the performance of the blockchain technology scheme designed in this paper in the intelligent review of access evidence. In the forensic scenario run by the algorithm in this paper, the stored evidence data is reduced by 1417 characters, the transaction response time is shortened by 175.361ms on average, and the block size is reduced by about 4 times. It proves that the blockchain algorithm scheme proposed in this paper can shrink the cost of depositing evidence, reduce the time of depositing evidence, and improve the efficiency of depositing evidence in the public security forensic system.
As the material foundation of language, speech is the basis for mastering language skills and capturing language information, and English learning must begin with the correct mastery of spoken language. Therefore, spoken language teaching occupies a rather important position in English teaching. In this study, we extract various features such as time-domain features and frequency-domain features from English spoken audio signals, use fuzzy logic inference model to represent each audio feature mapping as an affiliation function, and then optimize the parameters of the affiliation function by using adaptive neuro-fuzzy inference system, and solve the affiliation function to get the result of speech matching by the center of gravity method. Subsequently, a speech evaluation system is designed based on the speech matching model to assist intelligent spoken language teaching. The results of teaching practice show that students in the experimental class using the voice assessment system as a learning aid are significantly better than the control class in terms of speaking skills and learning attitudes (P<0.05). Through real-time feedback and personalized practice, the voice assessment system enables students to correct pronunciation errors immediately and gradually improve their speaking fluency and accuracy. It can also improve students' self-efficacy and learning motivation. This study confirms the effectiveness of the fuzzy logic-based audio classification and speech matching model in improving students' spoken English proficiency and reveals its potential for wide application in future spoken English education.
The continuous development of data analysis technology in the era of big data provides new methods for the analysis of college students’ ideological dynamic data, and also provides new ideas for the scientific construction of ideological and political education disciplines in colleges and universities. In this paper, based on the word frequency analysis, set up the keyword context, collect the keywords of the ideological dynamics of party members and students, extract and examine the feature vectors in them, put all the keyword feature vectors, form the keyword feature vector set, use the method of keyword vector research, carry out the descriptive and differential analysis of the survey data of the ideological dynamics of the party members’ development in the management of colleges and universities, and construct the party members’ ideological dynamics management mechanism based on the existing problems , analyzing the effect of management decisions in colleges and universities. The kurtosis values of the four indicators of party members’ political thoughts, learning thoughts, innovative thoughts and consumption thoughts are -1.4685, -0.4496, -0.9871 and -1.5614 respectively, which are on the low side, indicating that the performance of the respondents is more unified and concentrated in these four indicators, and laying a feasible foundation for the subsequent relevant analysis. In campus adaptation, self-efficacy, and life satisfaction, the number of party students who performed agreeably, correctly, and positively were 116, 202, and 142, respectively, and after the reform of college management decisions, the students’ performance in these three aspects changed.
Existing natural language generation models often face the problems of context loss and incoherent responses when dealing with multi-round dialogs. In this paper, a multi-round dialog system based on Transformer architecture is constructed, and an intention recognition algorithm is used to form a technical support for the construction of multi-round dialog system. And the attention adapter is introduced into the natural language generation module in the system, which utilizes contextual features to improve the performance of the natural language generation model. Semantic slot extraction experiments are carried out on the ATIS dataset, and the F1 values of the semantic slot extraction task and intention recognition task of the BERT multi-task natural language generation model with the addition of the attention mechanism are improved by 0.64% and 0.15%, respectively. The multi-round dialog system designed in this paper has a perplexity of 18.33, and the BLEU metrics are higher on orders 1-4 compared to other models. Manually evaluated in terms of syntactic semantic coherence, relevance, and information content, the system performs better. It shows that the natural language generation model incorporating the attention mechanism can effectively improve the application effect of the multi-round dialog system.
The pattern design of clothing appearance is one of the important links in clothing design, which makes an important contribution to the overall aesthetics and sales of clothing. As a product of computer technology, the development and application of graphic processing technology has been extended to various industries and fields of society, especially in the field of design with more extensive use. However, the current clothing pattern design is still too dependent on the designer, so this paper is based on pattern processing, combined with fractal algorithm and genetic algorithm to build a pattern generation algorithm for clothing pattern. And the quality of the generated pattern is optimized based on the anti-alignment algorithm, so as to improve the overall quality of the generated pattern. After testing, the real-time generation speed of the pattern generation algorithms for clothing patterns in this paper is greater than 15FPS, and from the subjective and objective points of view, the generated patterns have good quality to meet the needs of use. After the anti-alignment optimization of this paper’s algorithm in different error intervals in the number of pixels accounted for the percentage of screen pixels are the highest, are more than 99%, to further validate the optimization effect of this paper’s method. Finally, in the evaluation of the use of the algorithm, the testers have a high degree of satisfaction with the dimensions of this paper’s algorithm, respectively, 4.04, 3.98, 4.21 and 4.11, which shows that this paper’s algorithm can satisfy the practical needs and can realize the intelligent generation of clothing pattern design.
The convergence of constitutional fundamental rights and administrative enforcement power should pursue multiple legal values, which requires that the operation of the power therein should be more division of labor than cooperation, and that constraints and synergies should be given equal importance. The article will construct the basic constitutional rights and administrative power into the constitutional construction of the subject and the subject of the executive branch, the introduction of the evolution of the game theory to construct the constitutional construction of the subject and the subject of the executive branch of the evolution of the game model, and the design of the game model of the gain function, the replication of dynamic equations and ESS equilibrium point. The initial value of each parameter in the evolutionary game model is set, and the evolutionary stable point of administrative power is simulated by MATLAB software, and the influence of the reward and punishment allocation coefficients on the evolutionary results of the system is explored. When the system evolution stable point strategy is (0,0) and (1,1), the two sides of the game tend to the stable equilibrium state of active cooperation, strengthened regulation and strict supervision. When the reward distribution coefficient and the punishment distribution coefficient gradually increase, the two sides in the evolutionary game system tends to stabilize the point (1,1) the faster the rate will be. In the process of constructing the fundamental rights of the constitution, combining internal and external with the administrative rights list monitoring mechanism can realize the optimal restriction on the application of administrative rights and promote the orderly and stable operation of administrative power.
This topic is based on the perspective of diagnostic evaluation, formative new evaluation, summative evaluation, in-depth analysis of deep learning to help the intelligent development of ideological and political education. The initially formulated questionnaire was modified several times, and the questionnaire design task was finally completed, and the formal distribution of questionnaires began to obtain the data for this study. At this level, the empirical research method combining quantitative research and qualitative research is used to deeply analyze the current situation of the intelligent development of ideological and political education in colleges and universities under the perspective of deep learning, with a view to contributing to the intelligent development of ideological and political education in colleges and universities. The mean value of the five dimensions of ideological and political education to stimulate the effectiveness of active learning (A1), promote the degree of in-depth understanding (A2), deepen the effect of interactive participation (A3), enhance the ability of higher-order thinking (A4), and expand the quality of the transfer of the use of the quality of the five dimensions (A5) is higher than 3.55 points, in addition to the significant difference in the characteristics of the samples (gender, academic qualifications, major, political profile), and puts forward five paths for the development of the five development paths, which are aimed at helping to promote the new era of the intelligent development of Civic and Political Education.
Optimization problems usually involve multiple objectives, while fuzzy cognitive maps can effectively show the causal relationship between concepts, and the combination of the two can greatly advance the development of the education field. In this paper, we design a fuzzy cognition-based knowledge map for labor education courses and a multi-objective optimization model for labor education courses to optimize learners’ learning paths and recommend personalized exercises from multiple stages. Through teaching experiments and regression analysis, the teaching effect of the multi-objective optimization algorithm in labor education courses is evaluated. This paper borrows the k-means algorithm to classify learners into four clusters, and the algorithm provides learning path optimization for different clusters of learners in labor education courses. The exercise recommendation accuracy of this paper’s algorithm ranges from 0.91 to 0.97 and has better novelty and diversity recommendation performance. In the experimental class in the fuzzy cognitively oriented multi-objective optimization labor course, the learners’ labor scores improve faster and are about 3.8 points higher than those of the traditional teaching, and the regression results show that this paper’s model has a positive and positive effect on the teaching effect. The average satisfaction scores of this paper’s model in labor education courses for the friendliness of teaching aid, effectiveness of cognitive diagnosis method, usefulness of path optimization, and reasonableness of personalized recommendation of exercises are above 4.3, indicating that the model has practical application value in labor education courses.
Driven by big data, e-commerce platforms have accumulated massive user behavior data, which can be transformed into valuable information after cleaning and feature selection. This study analyzes users’ historical behavioral data on e-commerce platforms, constructs a gradient boosting decision tree prediction model based on user, product, category, and two-by-two interaction behavioral features, and extracts designed feature data from raw CSV data based on Hiv as the prediction basis of the model. At the same time, clustering analysis is performed based on the user’s purchasing behavior (dwell time, browsing frequency) to generate user profiles. The experimental results show that after 7 days, the purchase conversion rate of browsing, collecting, adding to cart and purchasing tends to 0. Therefore, the time window for purchase behavior prediction is chosen to be 7 days. In this paper, the prediction model is only trained to 20 epochs, and the Loss value converges to about 0.14, which shows a good training effect. The model has the best classification performance for user purchase behavior prediction, with precision, recall, and F1 values between 0.91 and 0.97. The clustering algorithm divides the user purchase behavior into four clusters, where cluster class 4 has the best user value. In summary, using the gradient boosting decision tree model, e-commerce platforms can more accurately predict user purchasing behavior, thus improving user experience and platform economic benefits.
Intangible cultural heritage is an important part of national culture, carrying rich historical information and cultural value. This paper mainly generates digital media art based on the characteristics of intangible cultural symbols through fractal geometry, and builds a digital media communication mode of intangible cultural art on the basis of dynamic texture, so as to realize the digital protection and inheritance of intangible cultural heritage. On the basis of Transformer network model, further combined with fractal geometry technology, inductive bias lacking in Transformer is introduced from the perspective of translation invariance and locality, and the dynamic texture generation method of digital media art combined with fractal geometry and Transformer model is formed in this paper. Experimental results show that the application of translation invariance and locality can increase the ODS, OIS and AP indexes by 17.17%, 27.72% and 25.38%, respectively. In the self-built Miao pattern data set, this method can generate dynamic texture features of Miao culture and art more completely and clearly. At the same time, this paper can create digital media art for Miao embroidery patterns through the generated results of this method, and improve the audience influence and satisfaction of intangible cultural heritage.
Deep reinforcement learning, as an advanced machine learning method, is capable of automatically learning optimal decision-making strategies in complex environments. The core objective of this paper is to apply deep reinforcement learning algorithms to SolidLab’s microcontroller programming in order to realize the intelligent control of the linear one-stage inverted pendulum system. The study takes the linear one-stage inverted pendulum produced by A Technology Company as the control object, and adopts the model-free control structure of the deep reinforcement learning algorithm to build the controller and conduct virtual simulation experiments. Comparing the experimental effects of LQR and DQN algorithms, the LQR algorithm is better than the DQN algorithm in stabilizing pendulum control of inverted pendulum. Accordingly, a balance controller based on the offline Q learning algorithm is further designed to realize the inverted pendulum stabilization in kind. After optimizing the design strategy, the inverted pendulum system can be rapidly stabilized within 0.9s when it is perturbed by a small angle of about 12°. It shows that the method in this paper can realize the intelligent control of the inverted pendulum system at the linear level.
The steady development of economy makes the number of high-rise buildings increasing, and the water supply and drainage system becomes an important part of high-rise building construction. The purpose of this paper is to explore the drainage efficiency and fire fighting efficiency of BIM and green new energy in water supply and drainage system. Revit Mep software is mainly used to design the fire fighting module in the drainage system of high-rise buildings by combining BIM technology and new energy. A simulation mathematical model of water supply and drainage efficiency (including fluid control equations, etc.) was designed, and simulation experiments were conducted on the fire treatment efficiency of green new energy fire protection technology. The investigators’ ratings of the evaluation indexes of the water supply and drainage system design of BIM synergistic green new energy fire protection technology ranged from 4.06 to 4.54, indicating the feasibility of the approach. When the building floor is 25 floors, the water supply and drainage efficiency of the system in this paper is 80.45% and 84.52%, respectively. The fire fighting simulation experiment shows that the green new energy fire fighting technology integrating BIM can reduce the temperature of the room to 200~500℃ in 5 minutes. In the experiment, the method can extinguish the fire quickly and the residual concentration of smoke after extinguishing can be reduced to the normal range.
The purpose of this paper is to explore the effectiveness of integrated energy electric energy substitution in agriculture in the environment of energy saving and emission reduction. The fuzzy clustering algorithm is used to divide different family clusters according to the energy saving and emission reduction ability based on the use of comprehensive energy in agricultural enterprises. Based on the exponential smoothing method and equivalent calorific value method, a prediction model of electric energy substitution efficiency was constructed. Combined with the ANP method, the evaluation indexes of agricultural electric energy substitution efficiency were weighted and graded. In this paper, 100 agricultural enterprises are divided into 5 clusters according to their energy saving and emission reduction ability, which are optimization level, managed level, development level, pressure-based level, and initial level. The model of this paper predicts that the consumption of terminal coal, oil, and electric energy of agricultural projects in 2027 is between 31663.68 and 7447.991.67 million tons of standard coal, and that the electric energy substitution in the same year can be up to 693,755.69 million tons of standard coal. The comprehensive scores of the first-level indicators of economic benefit, environmental benefit, and social benefit are 91.06, 91.26, and 92.01 in order, and the comprehensive efficiency grade is “Excellence”. To summarize the results, this study suggests increasing the investment of electric energy substitution equipment in agricultural production to promote the synergistic development of integrated energy system and electric energy substitution strategy.
Fintech has not only greatly improved the operational efficiency of banks by introducing cutting-edge technologies such as big data, artificial intelligence, and blockchain, but also posed new challenges to banks’ risk management. This paper uses Monte Carlo simulation to explore the impact of fintech on banks’ operational efficiency and risk management. A VaR data model is used to analyze the impact of fintech on the operational efficiency of three types of commercial banks: big five banks, joint-stock banks, and city commercial banks. The non-performing loan ratio of China MS Bank is used as the empirical object for quantitative analysis of bank risk management. Monte Carlo simulation is used to realize the VaR calculation of banks’ NPL ratio. The empirical analysis finds that the impact of fintech on the operational efficiency of all three types of commercial banks is relatively significant, but there are differences in direction and lag period. Meanwhile, FinTech increases banks’ NPL ratio. It shows that fintech has a negative impact on bank risk management, for this reason, this paper develops relevant strategies to deal with the risk challenges brought by fintech according to bank types.
This paper describes the teaching problems and methods that need to be solved for the innovation of education mode from the needs of teaching conditions, teacher strength, school-enterprise cooperation, etc., and puts forward the “three lines and four passes” education mode based on situational teaching workshop for geology majors. The evaluation index system is constructed for the quality of “three lines and four passes” education model, and various colleges and universities in a certain province are selected for empirical analysis to analyze the quality of education for students in the province from three aspects. In order to determine the contribution size of the 10 secondary indicators in the education quality evaluation index system, the method of determining the weights of the indicators by using principal component analysis was used to calculate the education quality indexes of the public higher vocational colleges and universities and private higher vocational colleges and universities. The K-means cluster analysis method was performed on the basis of the hierarchical cluster analysis method, and seven tiers were divided. The analysis results show that professional education, facilities and equipment, teacher-student cooperation, group competitions, practical exercises, talent cultivation and vocational training have a greater weight of 5% in the evaluation index system of public and private institutions. In conclusion, it is concluded that the “three lines and four passes” education model based on master craftsmen workshop proposed in this paper has a better teaching effect on the quality of education.
Robotic process automation (RPA) technology along with the rapid development of information technology is increasingly widely used in various industries. This paper mainly explores its application in the field of electric power, and utilizes RPA technology to improve the quality and efficiency of power marketing audit. In order to solve the data anomaly problem in the process of power marketing audit, this paper adopts K-means algorithm to cluster the anomalous data, and combines with the correlation calculation to realize the identification and monitoring of the anomalous data of power marketing audit. Applying RPA technology to intelligent power marketing audit, we learn the normal pattern of data by training the self-encoder network, and correct or reject the abnormal data monitored. Reinforcement learning is used to optimize the audit strategy of RPA technology, and the efficiency of the audit is improved by maximizing the cumulative rewards. The application of RPA technology significantly improves the efficiency and accuracy of the overdue prediction and the work order generation and dispatching in the electric power marketing audit, in which the average working time of the overdue prediction work is reduced by 94.92% after the application of RPA technology, the average accuracy is improved by 21.80%, and the average working time of the work order generation and dispatching process is reduced by 21.80%, and the average working time of the work order generation and dispatching process is improved by 21.80%. The average working time of work order generation and dispatching process is reduced by 97.99% and the average accuracy rate is increased by 14.54%. The application of RPA technology effectively improves the efficiency and quality of power marketing audit.
In this paper, the entire chord progression is added to the generation process through a bidirectional LSTM model, and the Skip-connection method is used to accelerate the convergence speed in all recursive layers except the first one. Different musical emotions are classified based on the Hevner emotion model, and features such as pitch, duration, and tempo of musical emotions are parameterized. The forward neural network is used to construct the music emotion classification model, and the gradient descent learning algorithm is used to algorithmically control the forward neural network model. At the same time, this paper explains the significant enhancement of college students’ self-identity by music aesthetic education based on neural network model from two perspectives: theoretical research and empirical analysis. The results show that the music generation and music emotion classification models constructed based on the neural network algorithm in this paper show good performance in the experiments. After applying the neural network model containing music generation and music emotion classification to music aesthetic education and counseling college students on self-identity, the mean score of self-identity scale of students in the experimental group increased from 50.83 to 88.56, with an improvement of 75.78%, and the results were significant at the 1% level. The effectiveness of this paper’s method in enhancing college students’ self-identity is fully demonstrated.
VTOL uavs combine the advantages of VTOL capability of multi-rotor uavs and efficient fixed-wing cruise, but they also face challenges in performance, including weak wind resistance when hovering, low flight mode conversion efficiency and high-altitude fluctuation during conversion. In view of this, this paper introduces a new type of composite wing UAV, namely lifting wing quadrotor. Compared with quadrotor UAV, it is unique in that it is equipped with a lifting wing installed at a special Angle, which effectively improves the range and load, and solves the problem of weak wind resistance in the hovering stage of tail-seat UAV, and can realize efficient transition flight. A longitudinal position controller based on TECS total energy control algorithm is designed according to the flight characteristics of the transition stage. The effectiveness of the dynamic model and controller design is verified by experiments. The results show that the control algorithm can effectively improve the flight stability of the lift-wing quadrotor during the transition stage.
The article evaluates and predicts the effectiveness of medical English teaching through stepwise regression analysis in multivariate analysis method. It constructs an analytical prediction model of medical English teaching evaluation based on multivariate regression analysis. After initially establishing the evaluation index system of medical English teaching, effective evaluation indexes are screened out through stepwise regression, an effective evaluation index system of medical English teaching is constructed, and multiple regression equations for the quality of medical English teaching (students’ performance in medical English) are established. The prediction model of medical English teaching quality is constructed by eliminating the influential factors and abnormal data that do not have significance through multiple linear regression analysis. Teaching quality prediction equations were constructed by choosing teaching content, teaching method, teaching organization, teaching expression, teaching attitude, and overall effect of teaching. Among them, teaching content and teaching expression were significant, and the final prediction model of medical English teaching quality was Y=0.1958+0.1142*teaching content+0.7232* teaching expression. The 79.26% of students’ medical English performance can be explained by the multivariate linear regression analysis model.
Dance is interpreted through human body movements, dance movements can express the thoughts and emotions of dancers, and whether the dance movements are standardized or not in the creation of dance drama determines the quality of the creation of dance drama. In this paper, with the support of artificial intelligence and information technology, based on image recognition technology, we carry out the optimization research of dance movement recognition for dance drama creation. In this study, the principle and process of image recognition technology are first studied in depth, and then the motion detection method for dance movements is analyzed considering the static state of the background of the dance drama. On this basis, the recognition optimization of dance movements is completed based on the deep convolutional embedding attention mechanism, and the evaluation method based on recognition optimization is proposed for the creation of dance drama. The embedding method in this paper improves 12% over the baseline method, with an OA of 98.65%, while the amount of participation and FLOPs increase slightly. And the score1 and score2 of this paper’s method are the highest, which indicates that this method obtains a high model accuracy while sacrificing less number of model parameters and computational complexity. In addition, the network model structure of this paper is more efficient compared to other network model results. In the recognition effect analysis, the correct recognition rate of six standard dance movements such as center of gravity transition, time step, square step, lock step, fixed step and others are above 80%, with high recognition accuracy and excellent model performance.
Dance drama performance is an important part of human civilization, which runs through the long history of human development. With the continuous improvement of people’s spiritual and cultural needs, the pursuit of dance theater performance has become more and more intense. In this paper, we start from the basic knowledge of fractal geometry, such as the theory, concept, dimension, and generation mechanism of fractal, to launch the research on the optimization of the spatial layout of dance drama performance. The main work of this paper involves the following aspects: (1) It focuses on the definition and characteristics of fractal geometry, and deeply studies the application of fractal geometry in the optimization of the spatial layout of dance drama performances. (2) The demand for dance drama performance space optimization is analyzed in terms of developmental changes and audience demand. It can be seen that the footprint of the dance performance continues to increase, but the use of space is gradually decreasing, and the audience has higher expectations for the stage performance, the demand for the development of the dance performance and the audience demand to promote the process of optimization of the space layout of the dance performance. (3) This paper adopts the left-right symmetrical presentation, fully considers the visual effect of the viewers, and constructs the optimized layout of dance drama performance space based on fractal geometry. (4) The optimized layout method of this paper is applied to the actual evaluation of the effect, in which the two groups A and B, located in the main audience area, have higher overall ratings, respectively 5.78 and 6.04, and the main audience area has a better perspective experience, so the overall experience is relatively better. The score of the viewer experience after optimization of the dance drama performance space layout in this paper is 5.715 points, which indicates that the viewers are satisfied with the effect of optimization of the layout in this paper, and the better results of the optimization of the dance drama performance space layout in this paper provide some reference for the dance drama performance space layout.
The stability of slopes is related to many factors, among which rainfall and water table fluctuation are the most common natural factors leading to slope damage. Based on a non-homogeneous slope, numerical simulation analysis using FLAC3D software and intensity discounting method was conducted in the article to discuss the stability of the slope under different morphologies, to explore the influence of diving surface height and pit water level line on the slope stability, to put forward the support scheme and to carry out the effect test. The analysis shows that the increase of slope gradient, step length and slope height negatively affects the slope stability, among which the effect of slope height is the most significant, and the stability coefficient decreases by 49.61% when the slope height increases by 60m under the action of groundwater. The height of diving surface and pit water level line are both inversely proportional to the slope stability, and the decrease of slope stability produced by the increase of both is 10.53% and 42.06%, respectively, and the latter’s influence on the slope safety coefficient is much larger than the former. In addition, the comprehensive landslide prevention and control program, which adopts the construction of drainage facilities, the selection of drainage scheme and the strengthening of support at the rock stratum interface area, effectively improves the safety coefficient of the slope.
Accelerating the formation of new-quality productivity is a must for transforming the mode of economic growth and realizing high-quality development. Taking the new quality productivity as the research perspective, this study selects the panel data of 30 provincial-level administrative regions in China from 2013 to 2023 as the research object, combines the regression analysis method to empirically analyze the influence paths between high-performance computing, the new quality productivity, and the economic policy innovation, and explores the role mechanism of the new quality productivity through the mediation effect analysis. The results show that the regression coefficients of high-performance computing on new quality productivity and economic policy innovation, as well as on new quality productivity and economic policy innovation, are all greater than 0 (p < 0.01), i.e., high-performance computing accelerates the formation of new quality productivity and further promotes the development of economic policy innovation. In the economically developed eastern region, this path of action is significant at the 1% level, and its driving effect of HPC accelerating new quality productivity on economic policy innovation is stronger, compared to the central, western and northeastern regions, which are significant at the 5% level. The article's analysis advances the understanding of the drivers of new quality productivity development and the effects, mechanisms, and regional differences of high-performance computing-enabled new quality productivity and economic policy innovation.
Under the rapid development of China’s infrastructure, concrete materials are widely used. Traditional concrete materials have defects such as poor compressive performance, and steel fibre concrete has a broad engineering application prospect. In this study, the compressive performance of steel fibre recycled concrete was analysed and tested using experiments such as cubic compression test and split tensile compression test. Subsequently, the compressive strength prediction model of steel fibre recycled concrete is constructed by combining the experimental test results and the uniaxial compression constitutive model, and the prediction effect of the model is analysed. The results show that when the volume rate of steel fibre admixture in recycled concrete is 1.2%, the compressive strength of recycled concrete is the highest under different loading conditions, indicating that the admixture of steel fibre can improve the compressive performance of recycled concrete. It was also found that the prediction error of the prediction model for the compressive strength of concrete under standard curing conditions and low-pressure conditions averaged 1.49% and 1.19%, which has good prediction effect. The compressive strength prediction model proposed in this paper can achieve reliable prediction of the compressive properties of steel fibre recycled concrete, which lays a foundation for the reasonable use of recycled concrete materials under different conditions in infrastructure projects.
Starting from the concept of digital media art, virtual reality technology is used to complete the design of the virtual simulation environment, and the interaction function of the virtual simulation environment is perfected through relevant development software. With the support of artificial intelligence technology, we propose a strategy to improve the visual expression of digital media art based on support vector machines, and we also design a detailed implementation process. The subjects of this study were selected to evaluate the design of the virtual simulation environment and the visual expressiveness enhancement strategy using the scale test method. The experimental group was significant in the dimensions of integration (P=0.005, T=1.553), immersion (P=0.007, T=2.693), interactivity (P=0.001, T=0.867), and virtuality (P=0.002, T=3.581) before and after the intervention, and it was concluded that Support Vector Machines have an enhancement effect on the visual expressiveness of the creation of digital media art.
Cross-border e-commerce refers to a kind of international commercial activity in which trading entities belonging to different customs borders reach transactions, make payments and settlements through e-commerce platforms, and deliver commodities through cross-border logistics to complete the transactions. Based on the relevant components of cross-border logistics cost and risk, this paper applies Markowitz, Capital Asset Pricing Model (CAPM), Value at Risk Model (VaRM), and Creditmetrics model to measure the risk of cross-border logistics, respectively. Through the cost measurement of cross-border logistics losses, a simplified logistics risk cost minimization model is derived. The model is applied to Guangxi’s cross-border logistics company M. Monte Carlo simulation is used to estimate the risk and cost of its cross-border logistics, respectively, and the probability of IRR>13.246% is simulated to be 32.963%, which indicates that the probability of cross-border logistics results exceeding the IRR of 13.246% given in the economic analysis is 32.963%. It can be seen in the logistics cost estimation that the mean value of the monthly logistics cost estimation of Company M is 2764000.564 yuan, and the standard deviation is 15126.36321 yuan, and after 3000 simulation operations, the logistics cost estimation has a 95% probability of falling on the interval [2572000 yuan, 2964000 yuan]. In response to the results of the simulation operations, a logistics risk and cost control strategy is proposed that is consistent with the long-term development of M Company.
In the process of airborne LiDAR point cloud cable line extraction, there are problems such as complex shape of the pole tower and high noise influence, which lead to low accuracy of cable line point cloud extraction. This paper proposes a cable line point cloud extraction and reconstruction method based on point cloud chunking processing, improved multidimensional filtering, and density clustering algorithm. Firstly, the point cloud filtering data processing technology, and its three key techniques of streamlining, filtering, and alignment in point cloud data preprocessing are introduced. Secondly, the overall point cloud is processed in chunks according to the direction of power lines. Then, on the basis of surface fitting algorithm, the idea of grid division is introduced to propose an improved multidimensional filtering algorithm with point cloud filtering. Finally, the cable line point cloud is accurately extracted by the given adaptive density clustering solution, and the method of this paper is tested and evaluated for accuracy based on the measured point cloud data. The results show that: using the algorithm to extract the cable line points of the integrated integrity rate of 95.9796% or more, a time can be realized in the successful extraction of the power line, in order to ensure the accuracy of the extraction at the same time to improve the extraction efficiency, the research in this paper can be for the intelligent inspection of the cable line to provide a good value of engineering applications.
Cables are widely used in power transmission, and the measurement of key dimensions of cables is an indispensable part of the cable preparation process to help ensure their quality. In this article, a handheld laser 3D scanner is used to collect 3D point cloud data of cable dimensions, and the point cloud is denoised by bilateral filtering algorithm and combined with the direct method of coarse alignment and the ICP method of fine alignment to realize the alignment of 3D point cloud data of cables. Then, the cable diameter coordinates are obtained by fitting the cylindrical surface of the cable size to realize the calculation of the cable diameter, and a residual network-based edge detection model of the cable insulation layer is proposed to improve the feature extraction capability of the cable 3D point cloud data through the hollow convolutional residuals and the spatial attention mechanism. For the effectiveness of the above method, the cable 3D point cloud data is quantitatively verified. The average accuracy of the cable diameter calculation based on cylindrical surface fitting is 0.025 m. The AP value of the cable insulation layer edge detection model constructed based on residual network is 0.816, and the error range of the calculation results is between -1.46% and 1.44% when the cable insulation layer thickness is calculated based on the cable insulation layer edge detection results. Learning and analyzing dimensional features of cable 3D point cloud data by deep learning training model can significantly improve the measurement accuracy and measurement efficiency of cable dimensional features, which can provide a guarantee for improving the safety of cable operation.
Higher vocational colleges and universities should realize the optimal allocation of teaching resources to provide the necessary guarantee for the improvement of talent cultivation quality. The study puts forward the evaluation index system of teaching resource allocation for teaching resource allocation in higher vocational education, constructs the multi-objective allocation optimization model of teaching resources on this basis, determines the index weights by using the objective combination assignment method combining the principal component analysis method and entropy weight method, and applies NSGA-II algorithm to solve the model. Simulation analysis is carried out with several higher vocational colleges and universities in a city as an example, and the allocation optimization results of multiple teaching resources in higher vocational colleges and universities are obtained. After the optimization of resource allocation, the utilization efficiency and allocation efficiency of teaching resources in each college and university as a whole have been improved by 16.6% and 3.4%, respectively, and all of them tend to be in the state of equilibrium of allocation. The constructed teaching resource allocation optimization model can realize the optimization of teaching resource allocation and promote the reasonable allocation and utilization efficiency of teaching resources in higher vocational education.
Common wealth is the essential requirement of socialism, and it is also the goal that countless people have been relentlessly pursuing for thousands of years, and people have never ceased to earnestly aspire to and relentlessly pursue for equal enjoyment and common wealth. This paper studies the impact of coastal wetland tourism resource protection on the realization of common wealth. It analyzes the tourism resources of coastal wetland from the aspects of economic value and resource protection and utilization strategy. On this basis, a multilevel regression model is used to analyze the impact between the two.The tourism economy of each coastal wetland developed rapidly in 2023, which increased by 65%~97% compared with 2015, implying that the conservation and utilization of tourism resources can lead to economic growth and promote economic development. In the multilevel model, resource protection strategy expenditure (0.070), ecological condition (0.265), strategy realization channel (0.053) and institutional trust (0.166) all show significant effects on the level of common wealth. While the regional level variables GDP per capita, provincial ecological level, and tourism resource utilization have unstable effects on the common wealth of urban residents. Based on the multilevel regression model, this study investigates the influence mechanism of tourism resources protection and utilization strategy on common wealth, which provides a basis for the full development of the positive effect of coastal wetland tourism resources protection and utilization strategy on the realization of common wealth.
In the era of digital imaging, the art of photography has undergone profound changes, in which the calculation method of temporal art and symbolic space has become the key to understanding this art form. This paper analyzes the temporal form of photographic art and designs the symbolic space in photographic art, using digital photography technology based on drone remote sensing combined with collage photography technology. And through a variety of calculation methods, the time consciousness and symbolic space of the creative works are quantitatively embodied. The resolution of the photographic works obtained by scanning drone photography and surround photography is 9.448 and 9.966mm respectively, and the error in plane and height is low. The use of collage technology to express different emotions is demonstrated by the audience’s recognition score of more than 4. Digital technology embodies the time consciousness and symbolic space of photography, “storytelling”, “composition and perspective”, “light and color”, with an average increase of 16.9%, 20.36%, and 13.06% respectively. The regression results show that “image capture and processing”, “post-processing”, “high resolution and color reproduction”, “autofocus”, “Digital Signal Processing” can all contribute to the time-conscious and symbolic spatial embodiment of photographic art at the 0.001 level.
1970-2025 CP (Manitoba, Canada) unless otherwise stated.