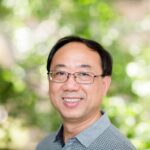
The main objective of this paper is to introduce a generalization of distance called superior distance in graphs. For two vertices \( u \) and \( v \) of a connected graph, we define \( \text{D}_{u,v} = \text{N}[u] \cup \text{N}[v] \). We define a \( \text{D}_{u,v} \)-walk as a \( u \)-\( v \) walk that contains every vertex of \( \text{D}_{u,v} \). The superior distance \( \text{d}_D(u,v) \) from \( u \) to \( v \) is the length of a shortest \( \text{D}_{u,v} \)-walk. In this paper, first we give the bounds for the superior diameter of a graph and a property that relates the superior eccentricities of adjacent vertices. Finally, we investigate those graphs that are isomorphic to the superior center of some connected graph and those graphs that are isomorphic to the superior periphery of some connected graph.
For any \( h \in \mathbb{N} \), a graph \( G = (V, E) \) is said to be \( h \)-magic if there exists a labeling \( l: E(G) \to \mathbb{Z}_h – \{0\} \) such that the induced vertex set labeling \( l^+: V(G) \to \mathbb{Z}_h \) defined by
$$l^+(v) = \sum_{uv \in E(G)} l(uv)$$
is a constant map. For a given graph \( G \), the set of all \( h \in \mathbb{Z}_+ \) for which \( G \) is \( h \)-magic is called the integer-magic spectrum of \( G \) and is denoted by \( IM(G) \). The concept of integer-magic spectrum of a graph was first introduced in [4]. But unfortunately, this paper has a number of incorrect statements and theorems. In this paper, first we will correct some of those statements, then we will determine the integer-magic spectra of caterpillars.
A sequence \( S \) is potentially \( K_{m}-C_4 \)-graphical if it has a realization containing a \( K_m-C_4 \) as a subgraph. Let \( \sigma(K_m-C_4,n) \) denote the smallest degree sum such that every \( n \)-term graphical sequence \( S \) with \( \sigma(S) \geq \sigma(K_m-C_4,n) \) is potentially \( K_m-C_4 \)-graphical. In this paper, we prove that \( \sigma(K_m-C_4,n) \geq (2m-6)n-(m-3)(m-2)+2 \), for \( n \geq m \geq 4 \). We conjecture that equality holds for \( n \geq m \geq 4 \). We prove that this conjecture is true for \( m = 5 \).
In 1975, Leech introduced the problem of finding trees whose edges can be labeled with positive integers in such a way that the set of distances (sums of weights) between vertices is \(\{1, 2, \dots, \binom{n}{2}\}\), where \(n\) is the number of vertices. We refer to such trees as perfect distance trees. More generally, we define a distinct distance tree to be a weighted tree in which the distances between vertices are distinct. In this article, we focus on identifying minimal distinct distance trees. These are the distinct distance trees on \(n\) vertices that minimize the maximum distance between vertices. We determine \(M(n)\), the maximum distance in a minimal distinct distance tree on \(n\) vertices, for \(n \leq 10\), and give bounds on \(M(n)\) for \(n \geq 11\). This includes a determination of all perfect distance trees for \(n < 18\). We then consider trees according to their diameter and show that there are no further perfect distance trees with diameter at most \(3\). Finally, generalizations to graphs, forests, and distinct distance sets are considered.
A bijection \( \lambda: V \cup E \cup F \to \{1, 2, 3, \dots, |V| + |E| + |F|\} \) is called a \( d \)-antimagic labeling of type \( (1, 1, 1) \) of plane graph \( G(V, E, F) \) if the set of \( s \)-sided face weights is \( W_s = \{a_s + a_s+d, a_s+2d, \dots, a_s + (f_s-1)d\} \) for some integers \( s \), \( a_s \), and \( d \), where \( f_s \) is the number of \( s \)-sided faces and the face weight is the sum of the labels carried by that face and the edges and vertices surrounding it. In this paper, we examine the existence of \( d \)-antimagic labelings of type \( (1, 1, 1) \) for a special class of plane graphs \( {C}_a^b \).
GWhD(\(v\))s, or Generalized Whist Tournament Designs on \( v \) players, are a relatively new type of design. GWhD(\(v\))s are (near) resolvable (\(v,k,k-1\)) BIBDs. For \( k = et \), each block of the design is considered to be a game involving \( e \) teams of \( t \) players each. The design is subject to the requirements that every pair of players appears together in the same game exactly \( t-1 \) times as teammates and exactly \( k-t \) times as opponents. These conditions are referred to as the Generalized Whist Conditions, and when met, we refer to the (N)RBIBD as a (\( t, k \)) GWhD(\(v\)). When \( k = 10 \), necessary conditions on \( v \) are that \( v \equiv 0, 1 \pmod{10} \). In this study, we focus on the existence of (\(2,10\)) GWhD(\(v\)), \(v \equiv 1 \pmod{10}\). It is known that a (\(2,10,9\))-NRBIBD does not exist. Therefore, it is impossible to have a (\(2,10\)) GWhD(\(21\)). It is established here that (\(2,10\)) GWhD(\(10n+1\)) exist for all other \(v\) with at most 42 additional possible exceptions.