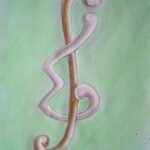
A simple acyclic graphoidal cover of a graph \( G \) is a collection \( \psi \) of paths in \( G \) such that every path in \( \psi \) has at least two vertices, every vertex of \( G \) is an internal vertex of at most one path in \( \psi \), every edge of \( G \) is in exactly one path in \( \psi \), and any two paths in \( \psi \) have at most one vertex in common. The minimum cardinality of a simple acyclic graphoidal cover of \( G \) is called the simple acyclic graphoidal covering number of \( G \) and is denoted by \( \eta_{as}(G) \). A simple acyclic graphoidal cover \( \psi \) of \( G \) with \( |\psi| = \eta_{as}(G) \) is called a minimum simple acyclic graphoidal cover of \( G \). Two minimum simple acyclic graphoidal covers \( \psi_1 \) and \( \psi_2 \) of \( G \) are said to be isomorphic if there exists an automorphism \( \alpha \) of \( G \) such that \( \psi = \{\alpha(P) : P \in \psi_1\} \). In this paper, we characterize trees, unicyclic graphs, and wheels in which any two minimum simple acyclic graphoidal covers are isomorphic.
In this paper, we study the domination number, the global domination number, the cographic domination number, the global cographic domination number, and the independent domination number of all the graph products which are non-complete extended \( p \)-sums (NEPS) of two graphs.
A sum composite labeling of a \((p,q)\) graph \( G = (V,E) \) is an injective function \( f : V(G) \to \{1,2,\dots,2p\} \) such that the function \( f^+ : E(G) \to C \) is also injective, where \( C \) denotes the set of all composite numbers and \( f^+ \) is defined by \( f^+(uv) = f(u) + f(v) \) for all \( uv \in E(G) \). A graph \( G \) is sum composite if there exists a sum composite labeling for \( G \). We give some classes of sum composite graphs and some classes of graphs which are not sum composite. We prove that it is possible to embed any graph \( G \) with a given property \( P \) in a sum composite graph which preserves the property \( P \), where \( P \) is the property of being connected, eulerian, hamiltonian, or planar. We also discuss the NP-completeness of the problem of determining the chromatic number and the clique number of sum composite graphs.
A \((p,q)\)-graph \( G \) is said to be \((k,d)\)-multiplicatively indexable if there exists an injection \( f : V(G) \to \mathbb{N} \) such that \( f^\times(E(G)) = \{k,k+d,\dots,k+(q-1)d\} \), where \( f^\times : E(G) \to \mathbb{N} \) is defined by \( f^\times(uv) = f(u)f(v) \) for every \( uv \in E(G) \). If further \( f(V(G)) = \{1,2,\dots,p\} \), then \( G \) is said to be a \((k,d)\)-strongly multiplicatively indexable graph. In this paper, we initiate a study of graphs that admit such labellings.
Public Key Cryptosystems (PKC) based on formal language theory and semi groups have been of interest and study. A PKC based on free group has been presented in [7]. Subsequently, another PKC using free partially commutative monoids and groups is studied in [1]. In this paper, we propose a PKC for chain cade pictures that uses a finitely presented group for encryption and free group for decryption. Also, we present another PKC for line pictures in the hexagonal grid, which uses a finitely presented group for encryption and finitely presented free partially commutative group for decryption.
Let \( G = (V, E) \) be a connected graph. A subset \( A \) of \( V \) is called an asteroidal set if for any three vertices \( u,v,w \) in \( A \), there exists a \( u \)-\( v \) path in \( G \) that avoids the neighbourhood of \( w \). The asteroidal chromatic number \( \chi_a \) of \( G \) is the minimum order of a partition of \( V \) into asteroidal sets. In this paper we initiate a study of this parameter. We determine the value of \( \chi_a \) for several classes of graphs, obtain sharp bounds, and Nordhaus-Gaddum type results.
Many approaches to drawing graphs in the plane can be formulated and solved as mathematical programming problems. Here, we consider only drawings of a graph where each edge is drawn as a straight-line segment, and we wish to minimize the number of edge crossings over all such drawings. Some formulations of this problem are presented that lead very naturally to other unsolved problems, some solutions, and some new open problems associated with drawing nonplanar graphs in the plane.
1970-2025 CP (Manitoba, Canada) unless otherwise stated.