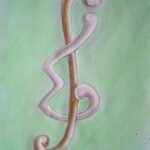
Given a graph \( G \), let \( E \) be the number of edges in \( G \). A \emph{vertex-magic edge labeling} of \( G \), defined by Wallis [12] in 2001, is a one-to-one mapping from the set of edges onto the set \(\{1, 2, \ldots, E\}\) with the property that at any vertex the sum of the labels of all the edges incident to that vertex is the same constant. In 2003, Hartnell and Rall [5] introduced a two-player game based on these labelings, and proved some nice results about winning strategies on graphs that contain vertices of degree one. In this paper, we prove results about winning strategies for certain graphs with cycles where the minimum degree is two.
A vertex labeling \( f: V \to \{0,1\} \) of the simple graph \( G = (V, E) \) induces a partial edge labeling \( f^*: E \to \{0,1\} \) defined by \( f^*(uv) = f(u) \) if and only if \( f(u) = f(v) \). Let \( v(i) \) and \( e(i) \) be the number of vertices and edges, respectively, that are labeled \( i \), and define the balance index set of \( G \) as \( \{|e(0) – e(1)| : |v(0) – v(1)| \leq 1\} \). In this paper, we determine the balance index sets of generalized wheels, which are the Zykov sum of a cycle with a null graph.
The channel assignment problem is the problem of assigning radio frequencies to transmitters while avoiding interference. This problem can be modeled and examined using graphs and graph colorings. \( L(2,1) \) coloring was first studied by Griggs and Yeh [6] as a model of a variation of the channel assignment problem. A no-hole coloring, introduced in [4], is defined to be an \( L(2,1) \) coloring of a graph which uses all the colors \(\{0,1,\ldots,k\}\) for some integer \(k\). An \( L(2,1) \) coloring is irreducible, introduced in [3], if no vertex labels in the graph can be decreased and yield another \( L(2,1) \) coloring. A graph \(G\) is inh-colorable if there exists an irreducible no-hole coloring on \(G\).
We consider the inh-colorability of bipartite graphs and Cartesian products. We obtain some sufficient conditions for bipartite graphs to be inh-colorable. We also find the optimal inh-coloring for some Cartesian products, including grid graphs and the rook’s graph.
Let \( G \) be a nontrivial connected graph of order \( n \) and \( k \) an integer with \( 2 \leq k \leq n \). For a set \( S \) of \( k \) vertices of \( G \), let \( \kappa(S) \) denote the maximum number \( \ell \) of pairwise edge-disjoint trees \( T_1, T_2, \ldots, T_\ell \) in \( G \) such that \( V(T_i) \cap V(T_j) = S \) for every pair \( i, j \) of distinct integers with \( 1 \leq i, j \leq \ell \). A collection \( \{T_1, T_2, \ldots, T_\ell\} \) of trees in \( G \) with this property is called a set of internally disjoint trees connecting \( S \). The \( k \)-connectivity \( \kappa_k(G) \) of \( G \) is defined as \( \kappa_k(G) = \text{min}\{\kappa(S)\} \), where the minimum is taken over all \( k \)-element subsets \( S \) of \( V(G) \). Thus \( \kappa_2(G) \) is the connectivity \( \kappa(G) \) of \( G \). In an edge-colored graph \( G \) in which adjacent edges may be colored the same, a tree \( T \) is a rainbow tree in \( G \) if no two edges of \( T \) are colored the same. For each integer \( \ell \) with \( 1 \leq \ell \leq \kappa_k(G) \), a \( (k, \ell) \)-rainbow coloring of \( G \) is an edge coloring of \( G \) (in which adjacent
Given a right-angled triangle of squares in a grid whose horizontal and vertical sides are \( n \) squares long, let \( N(n) \) denote the maximum number of dots that can be placed into the cells of the triangle such that each row, each column, and each diagonal parallel to the long side of the triangle contains at most one dot. It has been proven that \( N_f(n) = \lfloor \frac{2n+1}{3} \rfloor \). In this note, we give a new proof of the upper bound \( N_f(n) \leq \lfloor \frac{2n+1}{3} \rfloor \) using linear programming techniques.
In 1975, Leech introduced the problem of labeling the edges of a tree with distinct positive integers so that the sums along distinct paths in the tree were distinct, and the set of such path-sums were consecutive starting with one. We generalize this problem to labelings from arbitrary finite Abelian groups, with a particular focus on direct products of the additive group of \( \mathbb{Z}_2 \).
Let \( G \) be a simple graph. Any vertex labeling \( f: V(G) \to \mathbb{Z}_2 \) induces an edge labeling \( f^*: E(G) \to \mathbb{Z}_2 \) according to \( f^*(xy) = f(x) + f(y) \). For each \( i \in \mathbb{Z}_2 \), define \( v_f(i) = |\{v \in V(G) : f(v) = i\}| \), and \( e_f(i) = |\{e \in E(G) : f^*(e) = i\}| \). The friendly index set of the graph \( G \) is defined as \( \{|e_f(0) – e_f(1)| : |v_f(0) – v_f(1)| \leq 1\} \). We determine the friendly index sets of connected \( (p, p+1) \)-graphs with minimum degree \( 2 \). Many of them form arithmetic progressions. Those that are not miss only the second terms of the progressions.
1970-2025 CP (Manitoba, Canada) unless otherwise stated.