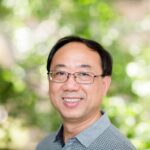
The spectrum for \(k\)-perfect \(3k\)-cycle systems is considered here for arbitrary \(k \not\equiv 0 \pmod{3}\). Previously, the spectrum when \(k = 2\) was dealt with by Lindner, Phelps, and Rodger, and that for \(k = 3\) by the current authors. Here, when \(k \equiv 1\) or \(5 \pmod{6}\) and \(6k + 1\) is prime, we show that the spectrum for \(k\)-perfect \(3k\)-cycle systems includes all positive integers congruent to \(1 \pmod{6k}\) (except possibly the isolated case \(12k + 1\)). We also complete the spectrum for \(k = 4\) and \(5\) (except possibly for one isolated case when \(k = 5\)), and deal with other specific small values of \(k\).
An efficient dominating set \(S\) for a graph \(G\) is a set of vertices such that every vertex in \(G\) is in the closed neighborhood of exactly one vertex in \(S\). If \(G\) is connected and has no vertices of degree one, then \(G\) has a spanning tree which has an efficient dominating set. An \(O(n)\) algorithm for finding such a spanning tree and its efficient dominating set is given.
Numbers similar to the van der Waerden numbers \(w(n)\) are studied, where the class of arithmetic progressions is replaced by certain larger classes. If \(\mathcal{A}’\) is such a larger class, we define \(w'(n)\) to be the least positive integer such that every \(2\)-coloring of \(\{1, 2, \ldots, w'(n)\}\) will contain a monochromatic member of \(\mathcal{A}’\). We consider sequences of positive integers \(\{x_1, \ldots, x_n\}\) which satisfy \(x_i = a_i x_{i-1} + b_i x_{i-2}\) for \(i = 3, \ldots, n\) with various restrictions placed on the \(a_i\) and \(b_i\). Upper bounds are given for the corresponding functions \(w'(n)\). Further, it is shown that the existence of somewhat stronger bounds on \(w'(n)\) would imply certain bounds for \(w(n)\).
For any graph \(G\), and all \(s = 2^k\), we show there is a partition of the vertex set of \(G\) into \(s\) sets \(U_1, \ldots, U_s\), so that both:
\(e(G[U_i]) \leq \frac{e(G)}{s^2} + \sqrt{\frac{e(G)}{s}}, \quad \text{for } i = 1, \ldots, s\) and \(\sum\limits_{i=1}^s e(G[U_i]) \leq \frac{e(G)}{s}.\)
The basic interpolation theorem states that if graph \(G\) contains spanning trees having \(m\) and \(n\) leaves, with \(m < n\), then for each integer \(k\) such that \(m < k < n\), \(G\) contains a spanning tree having \(k\) leaves. Various generalizations and related topics will be discussed.
We find the set of integers \(v\) for which \(\lambda K_v\) may be decomposed into sets of four triples forming Pasch configurations, for all \(\lambda\). We also remove the remaining exceptional values of \(v\) for decomposing \(K_v\) into sets of other four-triple configurations.
In this paper, we consider some combinatorial structures called balanced arrays (\(B\)-arrays) with a finite number of elements, and we derive some necessary conditions in the form of inequalities for the existence of these arrays. The results obtained here make use of the H\”{o}lder Inequality.
We present efficient algorithms for computing the matching polynomial and chromatic polynomial of a series-parallel graph in \(O(n^{3})\) and \(O(n^2)\) time respectively. Our algorithm for computing the matching polynomial generalizes and improves the result in \([13]\) from \(O(n^3 \log n)\) time for trees and the chromatic polynomial algorithm improves the result in \([18]\) from \(O(n^4)\) time. The salient features of our results are the following:
Our techniques for computing the graph polynomials can be applied to certain other graph polynomials and other classes of graphs as well. Furthermore, our algorithms can also be parallelized into NC algorithms.
Given positive integers \(p\) and \(q\), a \((p, q)\)-colouring of a graph \(G\) is a mapping \(\theta: V(G) \rightarrow \{1, 2, \ldots, q\}\) such that \(\theta(u) \neq \theta(v)\) for all distinct vertices \(u, v\) in \(G\) whose distance \(d(u, v) \leq p\). The \(p\)th order chromatic number \(\chi^{(p)}(G)\) of \(G\) is the minimum value of \(q\) such that \(G\) admits a \((p, q)\)-colouring. \(G\) is said to be \((p, q)\)-maximally critical if \(\chi^{(p)}(G) = q\) and \(\chi^{(p)}(G + e) > q\) for each edge \(e\) not in \(G\).
In this paper, we study the structure of \((2, q)\)-maximally critical graphs. Some necessary or sufficient conditions for a graph to be \((2, q)\)-maximally critical are obtained. Let \(G\) be a \((2, q)\)-maximally critical graph with colour classes \(V_1, V_2, \ldots, V_q\). We show that if \(|V_1| = |V_2| = \cdots = |V_k| = 1\) and \(|V_{k+1}| = \cdots = |V_q| = h \geq 1\) for some \(k\), where \(1 \leq k \leq q-1\), then \(h \leq h^*\), where
\[h^* = \max \left\{k, \min\{q – 1, 2(q – 1 – k)\}\right\}.\]
Furthermore, for each \(h\) with \(1 \leq h \leq h^*\), we are able to construct a \((2, q)\)-maximally critical connected graph with colour classes \(V_1, V_2, \ldots, V_q\) such that \(|V_1| = |V_2| = \cdots = |V_k| = 1\) and \(|V_{k+1}| = \cdots = |V_q| = h\).