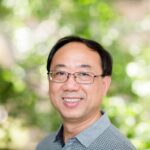
In this article, we define \(q\)-generalized Fibonacci polynomials and \(q\)-generalized Lucas polynomials using \(q\)-binomial coefficient and obtain their recursive properties. In addition, we introduce generalized \(q\)-Fibonacci matrix and generalized \(q\)-Lucas matrix, then we derive their basic identities. We define \((k,q,t)\)-symmetric generalized Fibonacci matrix and \((k,q,t)\)-symmetric generalized Lucas matrix, then we give the Cholesky factorization of these matrices. Finally, we give determinantal and permanental representations of these new polynomial sequences.
Stanley considered Dyck paths where each maximal run of down-steps to the \(x\)-axis has odd length; they are also enumerated by (shifted) Catalan numbers. Prefixes of these combinatorial objects are enumerated using the kernel method. A more challenging version of skew Dyck paths combined with Stanley’s restriction is also considered.
For \(r=1,2,…, 6\), we obtain generating functions \(F^{(r)}_{k}(y)\) for words over the alphabet \([k]\), where \(y\) tracks the number of parts and \([y^n]\) is the total number of distinct adjacent \(r\)-tuples in words with \(n\) parts. In order to develop these generating functions for \(1\le r\le 3\), we make use of intuitive decompositions but for larger values of \(r\), we switch to the cluster analysis method for decorated texts that was introduced by Bassino et al. Finally, we account for the coefficients of these generating functions in terms of Stirling set numbers. This is done by putting forward the full triangle of coefficients for all the sub-cases where \(r=5\) and 6. This latter is shown to depend on both periodicity and number of letters used in the \(r\)-tuples.
We consider the following variant of the round-robin scheduling problem: \(2n\) people play a number of rounds in which two opposing teams of \(n\) players are reassembled in each round. Each two players should play at least once in the same team, and each two players should play at least once in opposing teams. We provide an explicit formula for calculating the minimal numbers of rounds needed to satisfy both conditions. Moreover, we also show how one can construct the corresponding playing schedules.
Two binary structures \(\mathfrak{R}\) and \(\mathfrak{R’}\) on the same vertex set \(V\) are \((\leq k)\)-hypomorphic for a positive integer \(k\) if, for every set \(K\) of at most \(k\) vertices, the two binary structures induced by \(\mathfrak{R}\) and \(\mathfrak{R’}\) on \(K\) are isomorphic. A binary structure \(\mathfrak{R}\) is \((\leq k)\)-reconstructible if every binary
structure \(\mathfrak{R’}\) that is \((\leq k)\)-hypomorphic to \(\mathfrak{R}\) is isomorphic to \(\mathfrak{R}\). In this paper, we describe the pairs of \((\leq 3)\)-hypomorphic posets and the pairs of \((\leq 3)\)-hypomorphic bichains. As a consequence, we characterize the \((\leq 3)\)-reconstructible posets and the \((\leq 3)\)-reconstructible bichains. This answers a question suggested by Y. Boudabbous and C. Delhommé during a personal communication.
A tremendous amount of drug experiments revealed that there exists a strong inherent relation between the molecular structures of drugs and their biomedical and pharmacology characteristics. Due to the effectiveness for pharmaceutical and medical scientists of their ability to grasp the biological and chemical characteristics of new drugs, analysis of the bond incident degree (BID) indices is significant of testing the chemical and pharmacological characteristics of drug molecular structures that can make up the defects of chemical and medicine experiments and can provide the theoretical basis for the manufacturing of drugs in pharmaceutical engineering. Such tricks are widely welcomed in developing areas where enough money is lacked to afford sufficient equipment, relevant chemical reagents, and human resources which are required to investigate the performance and the side effects of existing new drugs. This work is devoted to establishing a general expression for calculating the bond incident degree (BID) indices of the line graphs of various well-known chemical structures in drugs, based on the drug molecular structure analysis and edge dividing technique, which is quite common in drug molecular graphs.
In this paper, we introduce a graph structure, called component intersection graph, on a finite dimensional vector space \(\mathbb{V}\). The connectivity, diameter, maximal independent sets, clique number, chromatic number of component intersection graph have been studied.
A linear system is a pair \((P,\mathcal{L})\) where \(\mathcal{L}\) is a finite family of subsets on a finite ground set \(P\) such that any two subsets of \(\mathcal{L}\) share at most one element. Furthermore, if for every two subsets of \(\mathcal{L}\) share exactly one element, the linear system is called intersecting. A linear system \((P,\mathcal{L})\) has rank \(r\) if the maximum size of any element of \(\mathcal{L}\) is \(r\). By \(\gamma(P,\mathcal{L})\) and \(\nu_2(P,\mathcal{L})\) we denote the size of the minimum dominating set and the maximum 2-packing of a linear system \((P,\mathcal{L})\), respectively. It is known that any intersecting linear system \((P,\mathcal{L})\) of rank \(r\) is such that \(\gamma(P,\mathcal{L})\leq r-1\). Li et al. in [S. Li, L. Kang, E. Shan and Y. Dong, The finite projective plane and the 5-Uniform linear intersecting hypergraphs with domination number four, Graphs and 34 Combinatorics (2018) , no.~5, 931–945.] proved that every intersecting linear system of rank 5 satisfying \(\gamma(P,\mathcal{L})=4\) can be constructed from a 4-uniform intersecting linear subsystem \((P^\prime,\mathcal{L}^\prime)\) of the projective plane of order 3 satisfying \(\tau(P^\prime,\mathcal{L}^\prime)=\nu_2(P^\prime,\mathcal{L}^\prime)=4\), where \(\tau(P^\prime,\mathcal{L}^\prime)\) is the transversal number of \((P^\prime,\mathcal{L}^\prime)\). In this paper, we give an alternative proof of this result given by Li et al., giving a complete characterization of these 4-uniform intersecting linear subsystems. Moreover, we prove a general case, that is, we prove if $q$ is an odd prime power and \((P,\mathcal{L})\) is an intersecting linear system of rank \((q+2)\) satisfying \(\gamma(P,\mathcal{L})=q+1\), then this linear system can be constructed from a spanning \((q+1)\)-uniform intersecting linear subsystem \((P^\prime,\mathcal{L}^\prime)\) of the projective plane of order \(q\) satisfying \(\tau(P^\prime,\mathcal{L}^\prime)=\nu_2(P^\prime,\mathcal{L}^\prime)=q+1\).
We classify all near hexagons of order \((3,t)\) that contain a big quad. We show that, up to isomorphism, there are ten such near hexagons.
Let \(G=(V,E)\) be a simple graph. A vertex \(v\in V(G)\) ve-dominates every edge \(uv\) incident to \(v\), as well as every edge adjacent to these incident edges. A set
\(D\subseteq V(G)\) is a vertex-edge dominating set if every edge of \(E(G)\) is ve-dominated by a vertex of \(D.\) The MINIMUM VERTEX-EDGE DOMINATION problem is to find a vertex-edge dominating set of minimum cardinality. A linear time algorithm to find the minimum vertex-edge dominating set for proper interval graphs is proposed. The vertex-edge domination problem is proved to be APX-complete for bounded-free graphs and NP-Complete for Chordal bipartite and Undirected Path graphs.