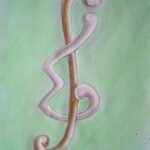
We consider a discrete-time dynamic problem in graphs in which the goal is to maintain a dominating set over an infinite sequence of time steps. At each time step, a specified vertex in the current dominating set must be replaced by a neighbor. In one version of the problem, the only change to the current dominating set is replacement of the specified vertex. In another version of the problem, other vertices in the dominating set can also be replaced by neighbors. A variety of results are presented relating these new parameters to the eternal domination number, domination number, and independence number of a graph.
The Turán number
Following a problem introduced by Schurch [M. Schurch,
A tree
A graph
Let
We are given suppliers and customers, and a set of tables. Every evening of the forthcoming days, there will be a dinner. Each customer must eat with each supplier exactly once, but two suppliers may meet at most once at a table. The number of customers and the number of suppliers who can sit together at a table are bounded above by fixed parameters. What is the minimum number of evenings to be scheduled in order to reach this objective? This question was submitted by a firm to the Junior company of a French engineering school some years ago. Lower and upper bounds are given in this paper, as well as proven optimal solutions with closed-form expressions for some cases.
In this paper, we mainly discuss the monotonicity of some sequences related to the hyperfibonacci sequences
The Ramsey number
We describe the construction of transitive
Gionfriddo and Lindner detailed the idea of the metamorphosis of
Eternal domination of a graph requires the positioning of guards to protect against an infinitely long sequence of attacks where, in response to an attack, each guard can either remain in place or move to a neighbouring vertex, while keeping the graph dominated. This paper investigates the
A graph
In this paper,
Let
As recognition of cordial graph is an NP-complete, it is interesting and significant to find the graphs whose arbitrary supersubdivision graphs are cordial. In this paper, we show that arbitrary supersubdivision of every bipartite graph is cordial. This result is obtained as a corollary of the general result that “Almost arbitrary supersubdivision of every graph is cordial”, where almost arbitrary supersubdivision is a relaxation of arbitrary supersubdivision graph.
Let
Under the conditions looser than previous works, this paper shows that the
In this paper,
The definition of
1970-2025 CP (Manitoba, Canada) unless otherwise stated.