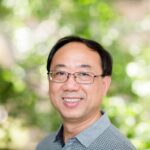
Let \(f(n)\) denote the number of essentially different factorizations of \(n\). In this paper, we prove that for every odd number \( > 1\), we have \(f(n) \leq c\frac{n}{\log n},\) where \(c\) is a positive constant.
A partition of the edge set of a hypergraph \(H\) into subsets inducing hypergraphs \(H_1,\ldots,H_r\) is said to be a \({decomposition}\) of \(H\) into \(H_1,\ldots,H_r\). A uniform hypergraph \(F = (\bigcup \mathcal{F}, \mathcal{F})\) is a \(\Delta\)-\({system}\) if there is a set \(K \subseteq V(F)\), called the \({kernel}\) of \(F\), such that \(A \cap B = K\) for every \(A, B \in \mathcal{F}\), \(A \neq B\). A disjoint union of \(\Delta\)-systems whose kernels have the same cardinality is said to be a \(constellation\). In the paper, we find sufficient conditions for the existence of a decomposition of a hypergraph \(H\) into:
a) \(\Delta\)-systems having almost equal sizes and kernels of the same cardinality,
b) isomorphic copies of constellations such that the sizes of their components are relatively prime.
In both cases, the sufficient conditions are satisfied by a wide class of hypergraphs \(H\).
The binding number of a graph \(G\) is defined to be the minimum of \(|N(S)|/|S|\) taken over all nonempty \(S \subseteq V(G)\) such that \(N(S) \neq V(G)\). In this paper, another look is taken at the basic properties of the binding number. Several bounds are established, including ones linking the binding number of a tree to the “distribution” of its end-vertices. Further, it is established that under some simple conditions, \(K_{1,3}\)-free graphs have binding number equal to \((p(G) – 1)/(p(G) – \delta(G))\) and applications of this are considered.
Strongly regular graphs are graphs in which every adjacent pair of vertices share \(\lambda\) common neighbours and every non-adjacent pair share \(\mu\) common neighbours. We are interested in strongly regular graphs with \(\lambda = \mu = k\) such that every such set of \(k\) vertices common to any pair always induces a subgraph with a constant number \(x\) of edges. The Friendship Theorem proves that there are no such graphs when \(\lambda = \mu = 1\). We derive constraints which such graphs must satisfy in general, when \(\lambda = \mu > 1\), and \(x \geq 0\), and we find the set of all parameters satisfying the constraints. The result is an infinite, but sparse, collection of parameter sets. The smallest parameter set for which a graph may exist has \(4896\) vertices, with \(k = 1870\).
The idea of a domino square was first introduced by J. A. Edwards et al. in [1]. In the same paper, they posed some problems on this topic. One problem was to find a general construction for a whim domino square of side \(n \equiv 3 \pmod{4}\). In this paper, we solve this problem by using a direct construction. It follows that a whim domino square exists for each odd side [1].
It is shown, through an exhaustive search, that there are no circulant symmetric Williamson matrices of order \(39\). The construction of symmetric but not circulant Williamson-type matrices of order \(39\), first given by Miyamoto, Seberry and Yamada, is given explicitly.
A graph \(G\) is defined by Chvátal \([4]\) to be \(n\) \({tough}\) if, given any set of vertices \(S, S \subseteq G\), \(c(G – S) \leq \frac{|S|}{n}\). We present several results relating to the recognition and construction of \(1\)-tough graphs, including the demonstration that all \(n\)-regular, \(n\)-connected graphs are \(1\)-tough. We introduce the notion of minimal \(1\)-tough graphs, and tough graph augmentation, and present results relating to these topics.
The integrity of a graph \(G\), denoted \(I(G)\), is defined by \(I(G) = \min\{|S| + m(G – S) : S \subset V(G)\}\) where \(m(G – S)\) denotes the maximum order of a component of \(G – S\); further an \(I\)-set of \(G\) is any set \(S\) for which the minimum is attained. Firstly some useful concepts are formalised and basic properties of integrity and \(I\)-sets identified. Then various bounds and interrelationships involving integrity and other well-known graphical parameters are considered, and another formulation introduced from which further bounds are derived. The paper concludes with several results on the integrity of circulants.
The new concept of \(M\)-structures is used to unify and generalize a number of concepts in Hadamard matrices, including Williamson matrices, Goethals-Seidel matrices, Wallis-Whiteman matrices, and generalized quaternion matrices. The concept is used to find many new symmetric Williamson-type matrices, both in sets of four and eight, and many new Hadamard matrices. We give as corollaries “that the existence of Hadamard matrices of orders \(4g\) and \(4h\) implies the existence of an Hadamard matrix of order \(8gh\)” and “the existence of Williamson type matrices of orders \(u\) and \(v\) implies the existence of Williamson type matrices of order \(2uv\)”. This work generalizes and utilizes the work of Masahiko Miyamoto and Mieko Yamada.
Consider \(n\) bridge teams each consisting of two pairs (the two pairs are called \({teammates}\)). A match is a triple \((i, j, b)\) where pair \(i\) opposes pair \(j\) on a board \(b\); here \(i\) and \(j\) are not teammates and “oppose” is an ordered relation. The problem is to schedule a tournament for the \(n\) teams satisfying the following conditions with a minimum number of boards:
We call a schedule satisfying the above five conditions a \({complete \; coupling \; round \; robin \; schedule}\) (CCRRS) and one satisfying the first four conditions a \({coupling \; round \; robin \; schedule}\) (CRRS). While the construction of CCRRS is a difficult combinatorial problem, we construct CRRS for every \(n \geq 2\).