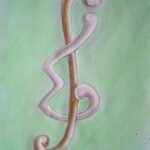
Certain graphs whose vertices are some collection of subsets of a fixed
We determine the minimal number of queries sufficient to find an unknown integer
A
Recently, there has been substantial interest in the problem of the spectrum of possible support sizes of different families of BIB designs. In this paper, we first prove some theorems concerning the spectrum of any
We obtain bounds for the separation number of a graph in terms of simpler parameters. With the aid of these bounds, we determine the separation number for various special graphs, in particular multiples of small graphs. This leads to concepts like robustness and asymptotic separation number.
A.M. Assaf, A. Hartman, and N. Shalaby determined in [1] the packing numbers
A hypergraph
Let
We consider the problem of finding the intersection points of a pencil of lines with rational slope on the
An orthogonal double cover of the complete graph
It is proved that an orthogonal double cover exists for all
The induced path number of a graph
Triple Youden rectangles are defined and examples are given. These combinatorial arrangements constitute a special class of
The Hall-condition number
One result here, that
The sum of a set of graphs
The main result of this study is that if
In this paper we study the existence of perfect Mendelsohn designs without repeated blocks and give several general constructions. We prove that for
A connected balanced bipartite graph
Let
A labeling (function) of a graph
In this thesis we examine the
The complete graph on
The minimal number of triples required to represent all quintuples on an
A set
We obtain an algorithm for finding
Special issue: Proceedings of International Conference on Discrete Mathematics (ICDM 2025)
1970-2025 CP (Manitoba, Canada) unless otherwise stated.