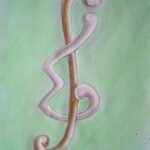
A Latin square is
In [13], we conjectured that if
In a finite projective plane, a
In this paper we determine the
A digraph obtained by replacing each edge of a complete
Let
Let
A generalized
1. For a new given parameter
2. Given the diameter and the maximum degree, the number of vertices is large enough.
For the first problem it is shown that if
For a graph
Some constructions of affine
We establish a connection between the principle of inclusion-exclusion and the union-closed sets conjecture. In particular, it is shown that every counterexample to the union-closed sets conjecture must satisfy an improved inclusion-exclusion identity.
Broadcasting in a network is the process whereby information, initially held by one node, is disseminated to all nodes in the network. It is assumed that, in each unit of time, every vertex that has the information can send it to at most one of its neighbours that does not yet have the information. Furthermore, the networks considered here are of bounded (maximum) degree
The path spectrum,
A graph
We study the behaviour of two domination parameters: the split domination number
A
The linear vertex-arboricity of a graph
The independence polynomial of graph
Balance has played an important role in the study of random graphs and matroids. A graph is balanced if its average degree is at least as large as the average degree of any of its subgraphs. The density of a non-empty loopless matroid is the number of elements of the matroid divided by its rank. A matroid is balanced if its density is at least as large as the density of any of its submatroids. Veerapadiyan and Arumugan obtained a characterization of balanced graphs; we extend their result to give a characterization of balanced matroids.
We show that there is a straight line embedding of the complete graph
An efficient algorithm for computing chromatic polynomials of graphs is presented. To make very large computations feasible, the algorithm combines the dynamic modification of a computation tree with a hash table to store information from isomorphically distinct graphs that occur during execution. The idea of a threshold facilitates identifying graphs that are isomorphic to previously processed graphs. The hash table together with thresholds allow a table look-up procedure to be used to terminate some branches of the computation tree. This table lookup process allows termination of a branch of the computation tree whenever the graph at a node is isomorphic to a graph that is stored in the hash table. The hashing process generates a large file of graphs that can be used to find any chromatically equivalent graphs that were generated. The initial members of a new family of chromatically equivalent graphs were discovered using this algorithm.
In this paper, we investigate the sufficient conditions for a graph to contain a cycle (path)
Let
For a graph
A graph
Let
Special issue: Proceedings of International Conference on Discrete Mathematics (ICDM 2025)
1970-2025 CP (Manitoba, Canada) unless otherwise stated.