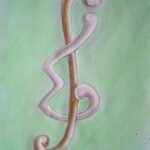
The sums
In a series of former papers, we obtained limit functions which describe scaling properties of the Farey sequence of order
Bulgarian solitaire is played on
For the special case
We introduce a polygonal cylinder
In this paper we find recurrence relations for the asymptotic probability a vertex is
Binomial coefficients of the form
In this paper, we analyze the stochastic properties of some large size (area) polyominoes’ perimeter such that the directed column-convex polyomino, the columnconvex polyomino, the directed diagonally-convex polyomino, the staircase (or parallelogram) polyomino, the escalier polyomino, the wall (orbargraph) polyomino. All polyominoes considered here are made of contiguous, not-empty columns, without holes, such that each column must be adjacent to some cell of the previous column. We compute the asymptotic (for large size n) Gaussian distribution of the perimeter, including the corresponding Markov property of the chain of columns, and the convergence to classical Brownian motions of the perimeter seen as a trajectory according to the successive columns. All polyominoes of size n are considered as equiprobable.
Convolution conditions are discussed for the
we discuss a framework for constructing large subsets of
Let
In this paper, we study a class of sequences of polynomials linked to the sequence of Bell polynomials. Some sequences of this class have applications on the theory of hyperbolic differential equations and other sequences generalize Laguerre polynomials and associated Lah polynomials. We discuss, for these polynomials, their explicit expressions, relations to the successive derivatives of a given function, real zeros and recurrence relations. Some known results are significantly simplified.
We show that if
This paper gives some new results on mutually orthogonal graph squares (MOGS). These generalize mutually orthogonal Latin squares in an interesting way. As such, the topic is quite nice and should have broad appeal. MOGS have strong connections to core fields of finite algebra, cryptography, finite geometry, and design of experiments. We are concerned with the Kronecker product of mutually orthogonal graph squares to get new results of the mutually orthogonal certain graphs squares.
For Cauchy numbers of the first kind
Let
Let
In 1998, D. Callan obtained a binomial identity involving the derangement numbers. In this paper, by using the theory of formal series, we extend such an identity to the generalized derangement numbers. Then, by using the same technique, we obtain other identities of the same kind for the generalized arrangement numbers, the generalized Laguerre polynomials, the generalized Hermite polynomials, the generalized exponential polynomials and the generalized Bell numbers, the hyperharmonic numbers, the Lagrange polynomials and the Gegenbauer polynomials.
In this paper, we present a method to construct a cyclic orthogonal double cover (CODC) of circulant graphs by certain kinds of coronas that model by linear functions.
Following the work of Cano and Díaz, we study continuous binomial coefficients and Catalan numbers. We explore their analytic properties, including integral identities and generalizations of discrete convolutions. We also conduct an in-depth analysis of a continuous analogue of the binomial distribution, including a stochastic representation as a Goldstein-Kac process.
In this paper, we introduce a new operator in order to derive some properties of homogeneous symmetric functions. By making use of the proposed operator, we give some new generating functions for
In this paper, we introduce the concept block matrix (B-matrix) of a graph
We consider analogs of several classical diophantine equations, such as Fermat’s last theorem and Catalan’s conjecture, for certain classes of analytic functions. We give simple direct proofs avoiding use of deep theorems in complex analysis. As a byproduct of our results, we obtain new proofs for the corresponding results over polynomials.
We define the
We also define the
For Cauchy numbers of the first kind
Let
Let
An \emph{Italian dominating function} on a digraph
A hypergraph
In this paper we prove that, there exists a regular bipartite self-complementary 3-uniform hypergraph
Neighborhood-prime labeling is a variation of prime labeling. A labeling
Let
For a finite simple graph
Let
Let
Rautenbach and Volkmann [Appl. Math. Lett. 20 (2007), 98–102] gave an upper bound for the
Addressing a problem posed by Chellali, Haynes, and Hedetniemi (Discrete Appl. Math. 178 (2014) 27–32), we prove
For a graph
These bounds on
Any dominating set of vertices in a triangle-free graph can be used to specify a graph coloring with at most one color class more than the number of vertices in the dominating set. This bound is sharp for many graphs. Properties of graphs for which this bound is achieved are presented.
A graph
Let
The
Let
If a tree
The decision problems of the existence of a Hamiltonian cycle or of a Hamiltonian path in a given graph, and of the existence of a truth assignment satisfying a given Boolean formula C, are well-known NP-complete problems. Here we study the problems of the uniqueness of a Hamiltonian cycle or path in an undirected, directed or oriented graph, and show that they have the same complexity, up to polynomials, as the problem U-SAT of the uniqueness of an assignment satisfying C. As a consequence, these Hamiltonian problems are NP-hard and belong to the class DP, like U-SAT.
A graph
In 1987, Alavi, Boals, Chartrand, Erdös, and Oellermann conjectured that all graphs have an ascending subgraph decomposition (ASD). In previous papers, we showed that all tournaments of order congruent to 1, 2, or 3 mod 6 have an ASD. In this paper, we will consider the case where the tournament has order congruent to 5 mod 6.
An
We introduce a new bivariate polynomial
which contains the standard domination polynomial of the graph
Let
In this paper, we consider the sequences
\end{abstract}
Let
Let
We consider inverse-conjugate compositions of a positive integer
1970-2025 CP (Manitoba, Canada) unless otherwise stated.