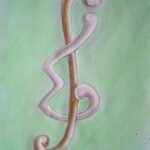
Suppose
Let
In this note, we shall show that the conjecture above holds for a class of graphs that includes the
A packing design (briefly packing) of order
Behzad has conjectured that a simple graph G can always be totally coloured using two more colours than the maximum degree in G. The conjecture has been verified for several special classes of graphs by Behzad, Chartrand and Cooper, Rosenfeld,
and Meyer, and by Vijayaditya for graphs with maximum degree less than or equal to 3.We show algorithmically that the conjecture is true for graphs with maximum degree 4.
The concept of self-complementary (s.c.) graphs is extended to almost self-complementary graphs. We define an
A triangulation of a surface is
The packing number
Ho and Shee [5] showed that for a graph
In this paper, we prove that there exists a cordial graph of order
Let
(ii) prove that if
prove that if
The notion of fusion in association schemes is developed and then applied to group schemes. It is shown that the association schemes derived in [1] and [4] are special cases of
Let
In this paper, it is proved that
In [1],[2], there are many assignment models. This paper gives a new assignment model and an algorithm for solving this problem.
Let
The fact that any
Here we prove a lower bound of
The problem of fairly dividing a piece of cake apparently originates with Hugo Steinhaus in 1948 at which time he raised the question of the number of cuts required in fair division algorithms. In this paper, an algorithm requiring
We study the lattice generated by the class of
In this paper, we study a problem related to one of the Turán problems: What is the maximum number of edges in a 3-graph without a complete subgraph on five vertices, the
If
It is shown that a symmetric design with
In this paper, we consider the structure of
In this paper, the authors study the vulnerability parameters of integrity, toughness, and binding number for two classes of graphs. These two classes of graphs are permutation graphs of complete graphs and permutation graphs of complete bipartite graphs
In this paper we examine bounds on
Three types of graphs are investigated with respect to cordiality, namely:graphs which are the complete product of two cordial graphs, graphs which are the subdivision graphs of cordial graphs, cactus graphs.
We give sufficient conditions for the cordiality of graphs of the first two types and show that a cactus graph is cordial if and only if the cardinality of its edge set is not congruent to
It is shown that there exists a 4-critical 3-uniform linear hypergraph of order
Essentially all pairs of forests
Steiner Heptagon Systems (SHS) of type 1, 2, and 3 are defined and the spectrum of type 2 SHSs (SHS2) is studied. It is shown that the condition
A graph is called well-covered if every maximal independent set has the same size. One generalization of independent sets in graphs is that of a fractional cover – attach nonnegative weights to the vertices and require that for every vertex the sum of all the weights in its closed neighbourhood be at least 1. In this paper, we consider and characterize fractionally well-covered graphs.
We prove that
In this paper, we prove that for any
We give a bijective proof for the identity
where
A bicover of pairs by quintuples of a
Special issue: Proceedings of International Conference on Discrete Mathematics (ICDM 2025)
1970-2025 CP (Manitoba, Canada) unless otherwise stated.