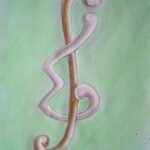
In this paper, we show that the independence polynomial \(I(G^*; x)\) of \(G^*\) is unimodal for any graph \(G^*\) whose skeleton \(G\) has stability number \(\alpha(G) \leq 8\). In addition, we show that the independence polynomial of \(K^*_{2,n}\) is log-concave with a unique mode.
Let \(G = (V,E)\) be a graph. A set \(S \subseteq V\) is a dominating set of \(G\) if every vertex not in \(S\) is adjacent to some vertex in \(S\). The domination number of \(G\), denoted by \(\gamma(G)\), is the minimum cardinality of a dominating set of \(G\). A set \(S \subseteq V\) is a total dominating set of \(G\) if every vertex of \(V\) is adjacent to some vertex in \(S\). The total domination number of \(G\), denoted by \(\gamma_t(G)\), is the minimum cardinality of a total dominating set of \(G\). In this paper, we provide a constructive characterization of those trees with equal domination and total domination numbers.
We consider a variation of a classical Turán-type extremal problem due to Bollobás \([2,p. 398, no. 13]\) as follows: determine the smallest even integer \(\sigma(C^k,n)\) such that every graphic sequence \(\pi = (d_1,d_2,\ldots,d_n)\) with term sum \(\sigma(\pi) = d_1 + d_2 + \cdots + d_n \geq \sigma(C^k,n)\) has a realization \(G\) containing a cycle with \(k\) chords incident to a vertex on the cycle. Moreover, we also consider a variation of a classical Turán-type extremal result due to Faudree and Schelp \([7]\) as follows: determine the smallest even integer \(\sigma(P_\ell,n)\) such that every graphic sequence \(\pi = (d_1,d_2,\ldots,d_n)\) with \(\sigma(\pi) \geq \sigma(P_\ell,n)\) has a realization \(G\) containing \(P_\ell\) as a subgraph, where \(P_\ell\) is the path of length 2. In this paper, we determine the values of \(\sigma(P_\ell,n)\) for \(n \geq \ell+1\) and the values of \(\sigma(C^k,n)\) for \(n \geq (k+3)(2k+5)\).
The hyperbolic Fibonacci function, which is the continuous extension of Binet’s formula for the Fibonacci number, transforms the Fibonacci number theory into a “continuous” theory because every identity for the hyperbolic Fibonacci function has its discrete analogy in the framework of the Fibonacci number. In this new paper, we define three important generalizations of the \(k\)-Fibonacci sine, cosine, and quasi-sine hyperbolic functions and then carry over many concepts and techniques that we learned in a standard setting for the \(k\)-Fibonacci sine, cosine, and quasi-sine hyperbolic functions to the generalizations of these functions.
A new construction of authentication codes with arbitration from pseudo-symplectic geometry over finite fields is given. The parameters and the probabilities of deceptions of the codes are also computed.
It was conjectured in a recently published paper that for any integer \(k \geq 8\) and any even integer \(n\) with \(2k+3 < n < 2k+\lfloor\frac{k}{2}\rfloor+3\), the \(k\)th power \(C_n^k\) of the \(n\)-cycle is not a divisor graph. In this paper, we prove this conjecture, hence obtaining a complete characterization of those powers of cycles which are divisor graphs.
Inspired by a recent paper by Giulietti, Korchmàros and Torres \([3]\), we provide equations for some quotient curves of the Deligne-Lusztig curve associated to the Suzuki group \(S_z(q)\).
In this paper, we study the global behavior of the nonnegative equilibrium points of the difference equation
\[x_{n+1} = \frac{ax_{n-2l}}{b+c\prod\limits_{i=0}^{k+1}x_{n-2i}}, \quad n=0,1,\ldots,\]
where \(a\), \(b\), and \(c\) are nonnegative parameters, initial conditions are nonnegative real numbers, and \(k\) and \(l\) are nonnegative integers, with \(l \leq k+1\).
The chromatic polynomial of a graph \(\Gamma\), \(C(\Gamma; \lambda)\), is the polynomial in \(\lambda\) which counts the number of distinct proper vertex \(\lambda\)-colorings of \(\Gamma\), given \(\lambda\) colors. By applying the addition-contraction method, chromatic polynomials of some sequences of \(2\)-connected graphs satisfy a number of recursive relations. We will show that by knowing the chromatic polynomial of a few small graphs, the chromatic polynomial of each of these sequences can be computed by utilizing either matrices or generating functions.
An \(f\)-coloring of a graph \(G\) is an edge-coloring of \(G\) such that each color appears at each vertex \(v \in V(G)\) at most \(f(v)\) times. The minimum number of colors needed to \(f\)-color \(G\) is called the \(f\)-chromatic index of \(G\). A simple graph \(G\) is of \(f\)-class 1 if the \(f\)-chromatic index of \(G\) equals \(\Delta_f(G)\), where \(\Delta_f(G) = \max_{v\in V(G)}\{\left\lceil\frac{d(v)}{f(v)}\right\rceil\}\). In this article, we find a new sufficient condition for a simple graph to be of \(f\)-class 1, which is strictly better than a condition presented by Zhang and Liu in 2008 and is sharp. Combining the previous conclusions with this new condition, we improve a result of Zhang and Liu in 2007.
We provide the specifics of how affine planes of orders three, four, and five can be used to partition the full design comprising all triples on \(9, 16\), and \(25\) elements, respectively. Key results of the approach for order five are generalized to reveal when there is potential for using suitable affine planes of order \(n\) to partition the complete sets of \(n^2\) triples into sets of mutually disjoint triples covering either all \(n^2\), or else precisely \(n^2 – 1\), elements.
In this paper, the notion of left-right and right-left \(f\)-derivation of a BCC-algebra is introduced, and some related properties are investigated. Also, we consider regular \(f\)-derivation and \(d\)-invariant on \(f\)-ideals in BCC-algebras.
Let \(G\) be a planar graph with maximum degree \(\Delta\). It’s proved that if \(\Delta \geq 5\) and \(G\) does not contain \(5\)-cycles and \(6\)-cycles, then \(la(G) = \lceil\frac{\Delta(G)}{2}\rceil\).
We call the digraph \(D\) an \(m\)-coloured digraph if the arcs of \(D\) are coloured with \(m\) colours. A subdigraph \(H\) of \(D\) is called monochromatic if all of its arcs are coloured alike.
A set \(N \subseteq V(D)\) is said to be a kernel by monochromatic paths if it satisfies the following two conditions:
(i) For every pair of different vertices \(u,v \in N\) there is no monochromatic directed path between them.
(ii) For every vertex \(x \in V(D) – N\), there is a vertex \(y \in N\) such that there is an \(xy\)-monochromatic directed path.
In this paper, it is proved that if \(D\) is an \(m\)-coloured \(k\)-partite tournament such that every directed cycle of length \(3\) and every directed cycle of length \(4\) is monochromatic, then \(D\) has a kernel by monochromatic paths.
Some previous results are generalized.
Let \(\mathcal{S}\) be a finite family of sets in \(\mathbb{R}^d\), each a finite union of polyhedral sets at the origin and each having the origin as an extreme point. Fix \(d\) and \(k\), \(0 \leq k \leq d \leq 3\). If every \(d+1\) (not necessarily distinct) members of \(\mathcal{S}\) intersect in a star-shaped set whose kernel is at least \(k\)-dimensional, then \(\cap\{S_i:S_i\in\mathcal{S}\}\) also is a star-shaped set whose kernel is at least \(k\)-dimensional. For \(k\neq 0\), the number \(d+1\) is best possible.
A graph is said to be cordial if it has a \(0-1\) labeling that satisfies certain properties. The second power of paths \(P_n^2\),is the graph obtained from the path \(P_n\) by adding edges that join all vertices \(u\) and \(v\) with \(d(u,v) = 2\). In this paper, we show that certain combinations of second power of paths, paths, cycles, and stars are cordial. Specifically, we investigate the cordiality of the join and the union of pairs of second power of paths and graphs consisting of one second power of path and one path and one cycle.
We initiate a study of the toughness of infinite graphs by considering a natural generalization of that for finite graphs. After providing general calculation tools, computations are completed for several examples. Avenues for future study are presented, including existence problems for tough-sets and calculations of maximum possible toughness. Several open problems are posed.
Let an \(H\)-point be a vertex of a tiling of \(\mathbb{R}^2\) by regular hexagons of side length 1, and \(D(n)\) a circle of radius \(n\) (\(n \in \mathbb{Z}^+\)) centered at an \(H\)-point. In this paper, we present an algorithm to calculate the number, \(\mathcal{N}_H(D(n))\), of H-points that lie inside or on the boundary of \(D(n)\). Furthermore, we show that the ratio \(\mathcal{N}_H(D(n))/n^2\) tends to \(\frac{2\pi}{S}\) as \(n\) tends to \(\infty\), where \(S = \frac{3\sqrt{3}}{2}\) is the area of the regular hexagonal tiles.
Let \(G\) be a finite, simple graph. We denote by \(\gamma(G)\) the domination number of \(G\). The bondage number of \(G\), denoted by \(b(G)\), is the minimum number of edges of \(G\) whose removal increases the domination number of \(G\). \(C_n\) denotes the cycle of \(n\) vertices. For \(n \geq 5\) and \(n \neq 5k + 3\), the domination number of \(C_5 \times C_n\) was determined in [6]. In this paper, we calculate the domination number of \(C_5 \times C_n\) for \(n = 5k + 3\) (\(k \geq 1\)), and also study the bondage number of this graph, where \(C_5 \times C_n\) is the cartesian product of \(C_5\) and \(C_n\).
A vertex cut that separates the connected graph into components such that every vertex in these components has at least \(g\) neighbors is an \(R^g\)-vertex-cut. \(R^g\)-vertex-connectivity, denoted by \(\kappa^g(G)\), is the cardinality of a minimum \(R^g\)-vertex-cut of \(G\). In this paper, we will determine \(\kappa^g\) and characterize the \(R^g\)-vertex-atom-part for the first and second type Harary graphs.
A graph \(G\) is supereulerian if \(G\) has a spanning eulerian subgraph. We use \(\mathcal{SL}\) to denote the families of supereulerian graphs. In 1995, Zhi-Hong Chen and Hong-Jian Lai presented the following open problem [2, problem 8.8]: Determine
\[L=\min\max\limits_{G\in SL-\{K_1\}}\{\frac{|E(H)|}{|E(G)|} : H \text{ is spanning eulerian subgroup of G}\}.\]
For a graph \(G\), \(O(G)\) denotes the set of all odd-degree vertices of \(G\). Let \(G\) be a simple graph and \(|O(G)| = 2k\). In this note, we show that if \(G\in{SL}\) and \(k \leq 2\), then \(L \geq \frac{2}{3}\).
It is known that the number of Dyck paths is given by a Catalan number. Dyck paths are represented as plane lattice paths which start at the origin \(O\) and end at the point \(P_n = (n,n)\) repeating \((1,0)\) or \((0,1)\) steps without going above the diagonal line \(OP_n\). Therefore, it is reasonable to ask of any positive integers \(a\) and \(b\) what number of lattice paths start at \(O\) and end at point \(A = (a, b)\) repeating the same steps without going above the diagonal line \(OA\). In this article, we show a formula to represent the number of such generalized Dyck paths.
Let \(G\) be a graph with vertex set \(V(G)\) and edge set \(E(G)\), and let \(A\) be an abelian group. A labeling \(f : V(G) \to A\) induces an edge labeling \(f^* : E(G) \to A\) defined by \(f^*(xy) = f(x) + f(y)\), for each edge \(xy \in E(G)\). For \(i \in A\), let \(v_f(i) = \mathrm{card}\{v \in V(G) : f(v) = i\}\) and \(e_f(i) = \mathrm{card}\{e \in E(G) : f^*(e) = i\}\). Let \(c(f) = \{|e_f(i) – e_f(j)|: (i, j) \in A \times A\}\). A labeling \(f\) of a graph \(G\) is said to be \(A\)-friendly if \(|v_f(i)- v_f(j)| \leq 1\) for all \((i, j) \in A \times A\). If \(c(f)\) is a \((0, 1)\)-matrix for an \(A\)-friendly labeling \(f\), then \(f\) is said to be \(A\)-cordial. When \(A = \mathbb{Z}_2\), the friendly index set of the graph \(G\), \(FI(G)\), is defined as \(\{|e_f(0) – e_f(1)| : \text{the vertex labeling } f \text{ is } \mathbb{Z}_2\text{-friendly}\}\). In [13] the friendly index set of cycles are completely determined. In this paper we describe the friendly index sets of cycles with parallel chords. We show that for a cycle with an arbitrary non-empty set of parallel chords, the numbers in its friendly index set form an arithmetic progression with common difference 2.
The eccentricity \(e(v)\) of a vertex \(v\) in a connected graph \(G\) is the distance between \(v\) and a vertex furthest from \(v\). The center \(C(G)\) is the subgraph induced by those vertices whose eccentricity is the radius of \(G\), denoted \(\mathrm{rad}G\), and the periphery \(P(G)\) is the subgraph induced by those vertices with eccentricity equal to the diameter of \(G\), denoted \(\mathrm{diam}G\). The annulus \(\mathrm{Ann}(G)\) is the subgraph induced by those vertices with eccentricities strictly between the radius and diameter of \(G\). In a graph \(G\) where \(\mathrm{rad}G < \mathrm{diam}G\), the interior of \(G\) is the subgraph \(\mathrm{Int}(G)\) induced by the vertices \(v\) with \(e(v) < \mathrm{diam}G\). Otherwise, if \(\mathrm{rad}G = \mathrm{diam}G\), then \(\mathrm{Int}(G) = G\). Another subgraph for a connected graph \(G\) with \(\mathrm{rad}G < \mathrm{diam}G\), called the exterior of \(G\), is defined as the subgraph \(\mathrm{Ext}(G)\) induced by the vertices \(v\) with \(\mathrm{rad}G < e(v)\). As with the interior, if \(\mathrm{rad}G = \mathrm{diam}G\), then \(\mathrm{Ext}(G) = G\). In this paper, the annulus, interior, and exterior subgraphs in trees are characterized.
This paper investigates the dihedral group as the array stabilizer of an augmented \(k\)-set of mutually orthogonal Latin squares. Necessary conditions for the stabilizer to be a dihedral group are established. A set of two-variable identities essential for a dihedral group to be contained in an array stabilizer are determined. Infinite classes of models that satisfy the identities are constructed.
A proper vertex coloring of a graph \(G = (V, E)\) is acyclic if \(G\) contains no bicolored cycle. A graph \(G\) is acyclically \(L\)-list colorable if for a given list assignment \(L = \{L(v) : v \in V\}\), there exists a proper acyclic coloring \(\phi\) of \(G\) such that \(\phi(v) \in L(v)\) for all \(v \in V(G)\). If \(G\) is acyclically \(L\)-list colorable for any list assignment with \(|L(v)| = k\) for all \(v \in V\), then \(G\) is acyclically \(k\)-choosable. In this paper, it is proved that every toroidal graph without 4- and 6-cycles is acyclically \(5\)-choosable.
The centro-polyhedral group \(\langle l,m,n\rangle\), for \(l, m, n \in \mathbb{Z}\), is defined by the presentation
\[\langle x, y, z : x^l = y^m = z^n = xyz \rangle.\]
In this paper, we obtain the periods of \(k\)-nacci sequences in centro-polyhedral groups and related groups.
We study two-path convexity in bipartite tournaments. For a bipartite tournament, we obtain both a necessary condition and a sufficient condition on the adjacency matrix for its rank to be two. We then investigate 4-cycles in bipartite tournaments of small rank. We show that every vertex in a bipartite tournament of rank two lies on a four cycle, and bipartite tournaments with a maximum number of 4-cycles do not necessarily have minimum rank.
A set \(S\) of vertices in a graph \(G\) is a clique dominating set of \(G\) if \(S\) contains at least one vertex of every clique \(C\) of \(G\). The clique domination number \(\gamma_q(G)\) and the upper clique domination number \(\gamma_q(G)\) are, respectively, the minimum and maximum cardinalities of a minimal clique dominating set of \(G\). In this paper, we prove that the problem of computing \(\Gamma_q(G)\) is NP-complete even for split graphs and the problem of computing \(\gamma_q(G)\) is NP-complete even for chordal graphs. In addition, for a block graph \(BG\) we show that the clique domination number is bounded above by the vertex independence number (\(\gamma_q(BG) \leq \beta(BG)\)) and give a linear algorithm for computing \(\gamma_q(BG)\).
Katerinis established the following result in [1]. Let \(G\) be a simple graph with \(\delta(G) \geq \lfloor\frac{|V(G)|}{2}+k\), where \(k\) is a non-negative integer. Let \(f : V(G) \to \mathbb{Z}^+\) be a function having the following properties:
(1) \(\frac{1}{2}\left({d_G(v) – (k+1)}{2}\right) \leq f(v) \leq \frac{1}{2}\left({d_G(v) + (k+1)}{2}\right)\) for every \(v \in V(G)\),
(2) \(\sum_{v\in V(G)} f(v) = |E(G)|\).
Then \(G\) has an orientation \(D\) such that \(d^+_D(v) = f(v)\), for every \(v \in V(G)\). In this paper, we focus on the sharpness of the above two inequalities.
A cosimple regular matroid \(M\) does not have disjoint circuits if and only if \(M \in \{M(K_{3,3}), M^*(K_n) \mid n \geq 3\}\). This extends a former result of Erdős and Pósa on graphs without disjoint circuits.
Suppose \(G\) is a finite graph with vertex-set \(V(G)\) and edge-set \(E(G)\). An \((a, d)\)-edge-antimagic total labeling on \(G\) is a one-to-one map \(f\) from \(V(G) \cup E(G)\) onto the integers \(1, 2, \ldots, |V(G)| + |E(G)|\) with the property that the edge-weights \(w(uv) = f(u) + f(v) + f(uv)\), \(uv \in E(G)\), form an arithmetic progression starting from \(a\) and having common difference \(d\). Such a labeling is called super if the smallest labels appear on the vertices. In this paper, we investigate the existence of super \((a, d)\)-edge-antimagic total labelings of disjoint union of multiple copies of complete bipartite graph.
Let \(G = (V, E)\) be a graph with no isolated vertex. A classical observation in domination theory is that if \(D\) is a minimum dominating set of \(G\), then \(V \setminus D\) is also a dominating set of \(G\). A set \(D’\) is an inverse dominating set of \(G\) if \(D’\) is a dominating set of \(G\) and \(D’ \subseteq V \setminus D\) for some minimum dominating set \(D\) of \(G\). The inverse domination number of \(G\) is the minimum cardinality among all inverse dominating sets of \(G\). The independence number of \(G\) is the maximum cardinality of an independent set of vertices in \(G\). Domke, Dunbar, and Markus (Ars Combin. \(72 (2004), 149-160)\) conjectured that the inverse domination number of \(G\) is at most the independence number of \(G\). We prove this conjecture for special families of graphs, including claw-free graphs, bipartite graphs, split graphs, very well covered graphs, chordal graphs, and cactus graphs.
A \(\lambda\)-design on \(v\) points is a set of \(v\) distinct subsets (blocks) of a \(v\)-set such that any two different blocks meet in exactly \(\lambda\) points and not all of the blocks have the same size. Ryser’s and Woodall’s \(\lambda\)-design conjecture states that every \(\lambda\)-design can be obtained from a symmetric design by a certain complementation procedure. A result of Ryser and Woodall establishes that there exist two integers, \(r\) and \(r^*\), such that each point in a \(\lambda\)-design is in exactly \(r\) or \(r^*\) blocks. The main result of the present paper is that the \(\lambda\)-design conjecture is true for \(\lambda\)-designs with \(\gcd(r-1,r^*-1)=7\).
Improving on Domokos’s improvement of Swan’s theorem, we show that under certain conditions on a finite digraph, whenever \(p,q\) are vertices, then the number of even Eulerian paths from \(p\) to \(q\) is the same as the number of odd ones from \(p\) to \(q\).
Double-loop networks have been widely studied as architecture for local area networks. A double-loop network \(G(N;s_1,s_2)\) is a digraph with \(N\) vertices \(0,1,\ldots,N-1\) and \(2N\) edges of two types:
\(s_1-edge\): \(i \rightarrow i+s_1 \pmod{N}\); \(i=0,1,\ldots,N-1\).
\(s_2-edge\): \(i \rightarrow i+s_2 \pmod{N}\); \(i=0,1,\ldots,N-1\).
for some fixed steps \(1 \leq s_1 < s_2 < N\) with \(\gcd(N,s_1,s_2) = 1\). Let \(D(N;s_1,s_2)\) be the diameter of \(G\) and let us define \(D(N) = \min\{D(N;s_1,s_2) | 1 \leq s_1 < s_2 < N \text{ and } gcd(N,s_1,s_2) = 1\}\), and \(D_1(N) = \min\{D(N;1,s) | 1 < s < N\}\). If \(N\) is a positive integer and \(D(N) < D_1(N)\), then \(N\) is called a non-unit step integer or a nus integer. Xu and Aguild et al. gave some infinite families of 0-tight nus integers with \(D_1(N) – D(N) \geq 1\). In this work, we give a method for finding infinite families of nus integers. As application examples, we give one infinite family of 0-tight nus integers with \(D_1(N) – D(N) \geq 5\), one infinite family of 2-tight nus integers with \(D_1(N) – D(N) \geq 1\) and one infinite family of 3-tight nus integers with \(D_1(N) – D(N) \geq 1\).
Ramanujan’s \(\mathop{_1\psi_1}\)-summation formula is one of the fundamental identities in basic hypergeometric series. We review proofs of this identity and clarify its connections with other basic hypergeometric series transformations and formulae. In particular, we shall put our main emphasis on methods that can be used not only to provide deeper insight into Ramanujan’s \(\mathop{_1\psi_1}\)-summation formula, but also to derive new transformations and identities for basic hypergeometric series.
A diagonalised lattice is a two dimensional grid, where we add exactly one arbitrary diagonal in each square, and color each vertex black or white.We show that for every diagonalised lattice there is a walk from the left to the right, using only black vertices, if and only if there is no walk from the top to the bottom, using only white vertices.
A terrace for \(\mathbb{Z}_m\) is an arrangement \((a_1, a_2, \ldots, a_m)\) of the \(m\) elements of \(\mathbb{Z}_m\) such that the sets of differences \(a_{i+1} – a_i\) and \(a_i – a_{i+1}\) (\(i = 1, 2, \ldots, m-1\)) between them contain each element of \(\mathbb{Z}_m \setminus \{0\}\) exactly twice. For \(m\) odd, many procedures are available for constructing power-sequence terraces for \(\mathbb{Z}_m\); each such terrace may be partitioned into segments one of which contains merely the zero element of \(\mathbb{Z}_m\) whereas each other segment is either (a) a sequence of successive powers of a non-zero element of \(\mathbb{Z}_m\) or (b) such a sequence multiplied throughout by a constant. For \(n\) an odd prime power satisfying \(n \equiv 1\) or \(3 \pmod{8}\), this idea has previously been extended by using power-sequences in \(\mathbb{Z}_n\) to produce some \(\mathbb{Z}_m\) terraces \((a_1, a_2, \ldots, a_m)\) where \(m = n+1 = 2^\mu\), with \(a_{i+1} – a_i = -(a_{i+1+\mu} – a_{i+\mu})\) for all \(i \in [1, \mu-1]\). Each of these “da capo directed terraces” consists of a sequence of segments, one containing just the element \(0\) and another just containing the element \(n\), the remaining segments each being of type (a) or (b) above with each of its distinct entries \(z\) from \(\mathbb{Z}_n \setminus \{0\}\) evaluated so that \(1 \leq x \leq n-1\). Now, for many odd prime powers \(n\) satisfying \(n \equiv 1 \pmod{4}\), we similarly produce narcissistic terraces for \(\mathbb{Z}_{n+1}\); these have \(a_{i+1} – a_i = a_{m-i+1} – a_{m-i}\) for all \(i \in [1, \mu-1]\).
We determine the full Sylow \(p\)-subgroup of the automorphism group of transitive \(k\)-ary relational structures of order \(p^2\), \(p\) a prime. We then find the full automorphism group of transitive ternary relational structures of order \(p^2\), for those values of \(p\) for which \({A_p}\) is the only doubly-transitive nonabelian simple group of degree \(p\). Finally, we determine optimal necessary and sufficient conditions for two Cayley \(k\)-ary relational structures of order \(p^2\), \(k < p\), to be isomorphic.
Let \(G\) be a finite group, \(S\) (possibly, contains the identity element) be a subset of \(G\). The Bi-Cayley graph \(\text{BC}(G, S)\) is a bipartite graph with vertex set \(G \times \{0,1\}\) and edge set \(\{\{(g,0), (gs,1)\}, g \in G, s \in S\}\). A graph \(X\) is said to be super-edge-connected if every minimum edge cut of \(X\) is a set of edges incident with some vertex. The restricted edge connectivity \(\lambda'(X)\) of \(X\) is the minimum number of edges whose removal disconnects \(X\) into nontrivial components. A \(k\)-regular graph \(X\) is said to be optimally super-edge-connected if \(X\) is super-edge-connected and its restricted edge connectivity attains the maximum \(2k-2\). In this paper, we show that all connected Bi-Cayley graphs, except even cycles, are optimally super-edge-connected.
1970-2025 CP (Manitoba, Canada) unless otherwise stated.