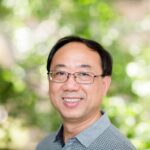
We classify all near hexagons of order \((3,t)\) that contain a big quad. We show that, up to isomorphism, there are ten such near hexagons.
Let \(G=(V,E)\) be a simple graph. A vertex \(v\in V(G)\) ve-dominates every edge \(uv\) incident to \(v\), as well as every edge adjacent to these incident edges. A set
\(D\subseteq V(G)\) is a vertex-edge dominating set if every edge of \(E(G)\) is ve-dominated by a vertex of \(D.\) The MINIMUM VERTEX-EDGE DOMINATION problem is to find a vertex-edge dominating set of minimum cardinality. A linear time algorithm to find the minimum vertex-edge dominating set for proper interval graphs is proposed. The vertex-edge domination problem is proved to be APX-complete for bounded-free graphs and NP-Complete for Chordal bipartite and Undirected Path graphs.
In this paper, we investigate the \((d,1)\)-total labelling of generalized Petersen graphs \(P(n,k)\) for \(d\geq 3\). We find that the \((d,1)\)-total number of \(P(n,k)\) with \(d\geq 3\) is \(d+3\) for even \(n\) and odd \(k\) or even \(n\) and \(k=\frac{n}{2}\), and \(d+4\) for all other cases.
By employing Kummer and Thomae transformations, we examine four classes of nonterminating \(_3F_2\)(1)-series with five integer parameters. Several new summation formulae are established in closed form.
Let \(G=(V,\,E)\) be a simple graph with vertex set \(V(G)\) and edge set \(E(G)\). The Lanzhou index of a graph \(G\) is defined by \(Lz(G)=\sum\limits_{u \in V(G)} d_u^2\overline{d}_u\), where \(d_u\) (\(\overline{d}_u \) resp.) denotes the degree of the vertex \(u\) in \(G\) (\(\overline{G}\), the complement graph of \(G\) resp.). It has predictive powers to provide insights of chemical relevant properties of chemical graph structures. In this paper we discuss some properties of Lanzhou index. Several inequalities having lower and upper bound for the Lanzhou index in terms of first, second and third Zagreb indices, radius of graph, eccentric connectivity index, Schultz index, inverse sum indeg index and symmetric division deg index, are discussed. At the end the Lanzhou index of corona and join of graphs have been derived.
We define an extremal \((r|\chi)\)-graph as an \(r\)-regular graph with chromatic number \(\chi\) of minimum order. We show that the Turán graphs \(T_{ak,k}\), the antihole graphs and the graphs \(K_k\times K_2\) are extremal in this sense. We also study extremal Cayley \((r|\chi)\)-graphs and we exhibit several \((r|\chi)\)-graph constructions arising from Turán graphs.
A dominating broadcast of a graph \(G\) is a function \(f : V(G) \rightarrow \lbrace 0, 1, 2, \dots ,\text{diam}(G)\rbrace\) such that \(f(v) \leqslant e(v)\) for all \(v \in V(G)\), where \(e(v)\) is the eccentricity of \(v\), and for every vertex \(u \in V(G)\), there exists a vertex \(v\) with \(f(v) > 0\) and \(\text{d}(u,v) \leqslant f(v)\). The cost of \(f\) is \(\sum_{v \in V(G)} f(v)\). The minimum of costs over all the dominating broadcasts of \(G\) is called the broadcast domination number \(\gamma_{b}(G)\) of \(G\). A graph $G$ is said to be radial if \(\gamma_{b}(G)=\text{rad}(G)\). In this article, we give tight upper and lower bounds for the broadcast domination number of the line graph \(L(G)\) of \(G\), in terms of \(\gamma_{b}(G)\), and improve the upper bound of the same for the line graphs of trees. We present a necessary and sufficient condition for radial line graphs of central trees, and exhibit constructions of infinitely many central trees \(T\) for which \(L(T)\) is radial. We give a characterization for radial line graphs of trees, and show that the line graphs of the \(i\)-subdivision graph of \(K_{1,n}\) and a subclass of caterpillars are radial. Also, we show that \(\gamma_{b}(L(C))=\gamma(L(C))\) for any caterpillar \(C\).
In this paper we introduce the concept of independent fixed connected geodetic number and investigate its behaviours on some standard graphs. Lower and upper bounds are found for the above number and we characterize the suitable graphs achieving these bounds. We also define two new parameters connected geo-independent number and upper connected geo-independent number of a graph. Few characterization and realization results are formulated for the new parameters. Finally an open problem is posed.
Let \(E(H)\) and \(V(H)\) denote the edge set and the vertex set of the simple connected graph \(H\), respectively. The mixed metric dimension of the graph \(H\) is the graph invariant, which is the mixture of two important graph parameters, the edge metric dimension and the metric dimension. In this article, we compute the mixed metric dimension for the two families of the plane graphs viz., the Web graph \(\mathbb{W}_{n}\) and the Prism allied graph \(\mathbb{D}_{n}^{t}\). We show that the mixed metric dimension is non-constant unbounded for these two families of the plane graph. Moreover, for the Web graph \(\mathbb{W}_{n}\) and the Prism allied graph \(\mathbb{D}_{n}^{t}\), we unveil that the mixed metric basis set \(M_{G}^{m}\) is independent.
Consider a total labeling \(\xi\) of a graph \(G\). For every two different edges \(e\) and \(f\) of \(G\), let \(wt(e) \neq wt(f)\) where weight of \(e = xy\) is defined as \(wt(e)=|\xi(e) – \xi(x) – \xi(y)|\). Then \(\xi\) is called edge irregular total absolute difference \(k\)-labeling of \(G\). Let \(k\) be the minimum integer for which there is a graph \(G\) with edge irregular total absolute difference labeling. This \(k\) is called the total absolute difference edge irregularity strength of the graph \(G\), denoted \(tades(G)\). We compute \(tades\) of \(SC_{n}\), disjoint union of grid and zigzag graph.